Journal of Infectious Diseases and Epidemiology
Essential Transition Metal Ion Complexation as a Strategy to Improve the Antimicrobial Activity of Organic Drugs
Graciela Borthagaray1, Melina Mondelli2 and María H Torre2*
1Microbiología (BIOCLIN), Facultad de Química, Universidad de la República, Uruguay
2Química Inorgánica (DEC), Facultad de Química, Universidad de la República, Uruguay
*Corresponding author:
María H Torre, Química Inorgánica (DEC), Facultad de Química, Universidad de la República, Gral. Flores 2124, CC1157, Montevideo, Uruguay, E-mail: mtorre.quim@gmail.com
J Infect Dis Epidemiol, JIDE-2-014, (Volume 2, Issue 2), Review Article; ISSN: 2474-3658
Received: February 26, 2016 | Accepted: April 30, 2016 | Published: May 02, 2016
Citation: Borthagaray G, Mondelli M, Torre MH (2016) Essential Transition Metal Ion Complexation as a Strategy to Improve the Antimicrobial Activity of Organic Drugs. J Infect Dis Epidemiol 2:014. 10.23937/2474-3658/1510014
Copyright: © 2016 Borthagaray G, et al. This is an open-access article distributed under the terms of the Creative Commons Attribution License, which permits unrestricted use, distribution, and reproduction in any medium, provided the original author and source are credited.
Abstract
In order to maintain the capacity to treat infections it is necessary to adopt strategies for the development of new drugs. The use of metal ions in medicine for their antimicrobial effects was prevalent for many years before the advent of antibiotics. During the second half of the twentieth century, active metal complexes with different chemical, physicochemical and biochemical properties than the constituents reborn by the threat posed by microbial multidrug resistance.
In this review, essential transition metal drugs with Cu(II), Zn(II) and Fe(III), showing known chemical structure, antimicrobial activities better than the free ligands and reported in the last ten years, are presented.
The scientific information realizes many metal complexes having antimicrobial activity, which covers Gram negative and Gram positive bacteria, mycobacteria, yeast and fungi and many of them would be good candidates for further pharmaceutical studies.
For these reasons the strategy of metal coordination is a potent tool to get numerous and diverse new antimicrobial agents.
Introduction
The research for new and better antimicrobial compounds is an important field due to different factors. The use of invasive medical procedures and extensive surgeries, the increase of immune compromised patients, among others, can affect the incidence of infections. Another big problem associated is that treatment of infectious diseases is being seriously hampered by the emergence and spread of microbial resistance. While the majority of human pathogens remain sensitive to antimicrobials, multi drug resistant (MDR) pathogens have emerged, being antimicrobial use the driving force behind their appearance. Frequently MDR bacteria are common cause of hospital infections, causing some community acquired infections too, and some strains are resistant to all current available antimicrobial. This phenomenon is the result of the microorganism ability to find a quick response to overcome an adverse environment through mutation and acquisition of new genes [1].
In order to maintain the capacity to treat infections it is necessary to adopt strategies that prevent or delay the development of resistance and preserve the efficiency of actual or new antimicrobials and the development of new drugs. The strategies proposed include the reduction of antimicrobials demand, through vaccination, improving water and sanitation and a supply of food free from bacterial pathogens; the development of new ways to treat infectious, like antibiotic cycling, finding agents that renew the antibacterial currently in use and bacteriophages therapy; and the search for new antimicrobials to treat low but growing numbers of MDR microbial infections [1].
With this aim, organic chemists have synthesized analogues and modifications or derivatives of existing organic compounds [2] or have researched on novel structures which microorganisms have never been exposed. This is the case of bedaquiline, the first drug in a new class of anti-Tuberculosis (TB) medications to be approved in more than 40 years by the US Food and Drug Administration [3].
Other strategies are the development of inhibitors that are co-administrated with antibiotics to neutralize resistance mechanisms; the search for natural occurring compound in bacteria, in plants, in the marine world and through the novel growth of uncultured microorganism; the search for synergistic action between older antimicrobials and between antimicrobial and essential oils; the search of entire genomes for new antibacterial targets; and new formulations of metals-based compounds for use as antimicrobial agents alterative to antibiotics. This last strategy is of interest in this report [4-7].
The use of metal compounds in medicine for their antimicrobial effects was prevalent for many years before the advent of antibiotics. These antibiotics were almost exclusively based on synthetic and natural organic products. Despite the recognition of the pharmacological properties of metal ions, scarce attention has been given to inorganic substances. However, during the second half of the twentieth century the use of molecules or ions coordinated with metals reborn by the threat posed by microbial MDR and became a good tool to provide new pharmacological active molecules [8].
This strategy was used in several areas of the pharmacology. In spite of the fact that metal-based drugs represent a relatively small percentage of total drugs, they fulfill important roles in medicine. Just consider the cisplatin (platinum-containing anti-cancer drug), the aurothioglucose (chrysotherapy for the treatment of rheumatoid arthritis) and the Ag-sulfadiazine (silver complex with antibacterial activity used in burns) to realize the importance of this field [9-11].
These complexes consist of a central ion and a surrounding array of ligands defined as molecules or ions that have electrons available for donating towards a positively charged species such as metal ions, forming dative covalent bonds [12]. These ligands can be pharmacological active molecules and the coordination with metals can modify the activity or they can be inactive but the complex acquires activity.
The complexes can present different chemical, physicochemical and biochemical properties than the constituents (central metal and ligands). The biological effectiveness does not depend solely on the release of metal ion or the active ligand, but it is associated rather strongly on the nature and structure of the new compound to which the metal ion is bound. The size, charge distribution, shape and redox potentials of complexes are essential properties related to the mechanism of action [13].
In the research of new antimicrobial agents there are several studies about new metal-based complexes active against bacteria and fungus. The mechanisms of action are different depending on the complex. They can act against the microorganism by the occupation of surface sites, which would normally be utilized in the initiation of the infection of the host cell preventing the first step in the infection. Alternatively, the compound may penetrate the cell wall, disturbing different molecule targets [13].
In this report, the purpose is to present different examples of essential transition metal drugs with Cu(II), Zn(II) and Fe(III). The selected metals with antimicrobial activity, reported many years ago [14], and the metal complexes chosen were those with known chemical structure, presenting antimicrobial properties better than the free ligand and reported in the last ten years. In the bibliography, it can be found works comparing the antimicrobial activity of complexes with a specific ligand and different metal ions and, on the opposite, studies of complexes with the same metal ion and a series of structural related ligands. In the first case and with the aim to rigorously study the influence of the metal ion, it is important to compare compounds with the same stoichiometry and similar structure.
This work provides information to understand the activity rather than attempt an exhaustive listing of metal-based antimicrobial molecules. For the sake of clarity we will present the different antimicrobial compounds grouped by metals, and for each metal we selected some examples to perform a more detailed discussion. The reported Minimum Inhibitory Concentration (MIC) was expressed in the units used by the authors in bibliographic references.
Cu(II) Compounds
Copper is an essential trace element for many biological functions. Free copper ion is reported to have toxic effects against bacteria and fungus [14].
Due to this behavior, numerous researchers used the copper coordination of organic molecules to improve the antimicrobial activity. In table 1, a list of novel copper compounds with antimicrobial activity is reported. All their ligands presented antimicrobial activity but the complexes were more active, while the free copper ion is inactive in the studied concentration.
Table 1: Copper complexes with antimicrobial activity.
View Table 1
Comparing the copper complexes with different ligands, we observed that the better activity was found for the heteroleptic complexes with CQ or ciprofloxacin against Gram negative and Gram positive bacteria, and especially the complex with ciprofloxacin against M. tuberculosis. Moreover, the copper complex with sulfamethoxazole presented good activity against S. aureus.
The comparative antimicrobial behavior of a series of copper complexes with heterocyclic sulfonamides having pyrimidine group (sulfadiazine, sulfamerazine, sulfamethazine), pyridine (sulfapyridine), pyridazine (sulfachloropyridazine, sulfamethoxypyridazine), isoxazole (sulfisoxazole, sulfamethoxazole) and diazomethizole (sulfamethizole) allowed to propose possible mechanisms of action [20].
These complexes were synthesized with the sulfonamide ligands shown in figure 1. The general synthesis method, represented in figure 2, is by mixing 2:1 sodium sulfonamide (pH = 9-10):CuSO4⋅5H2O. After stirring the precipitate formed was filtered, washed with water, dried at room temperature and protected from light.
These compounds are extensively used in medicine due to their antimicrobial properties and they present coordination versatility acting as monodentate ligands through the 4N or Nh atoms; as bidentate through the 1N and Nh or bridging two metal ions through 4N and Nh; as bidentate to one Cu(II) through Nh and 1N and bridging to an adjacent Cu(II) through the 4N [31,32].
As it is well known sulfonamides interfere with the use of p-aminobenzoic acid (PABA) in the biosynthesis of tetrahydrofolic acid, which is essential to bacterial metabolism. Modified toxicological and pharmacological properties have been observed when some of these sulfonamides are administered in the form of their metal complexes [20].
This study shows that all the complexes were active against S. aureus and E. coli but only the complexes with ligands having a five-membered heterocycle [Cu(sulfisoxazole)2(H2O)4]⋅2H2O, [Cu(sulfamethizole)2]⋅H2O, [Cu(sulfamethoxazole)2(H2O)4]⋅3H2O and [Cu(sulfamethoxazole)2]⋅H2O were more active than the free sulfonamides. None of the copper sulfate dilutions, used as controls, inhibited the growth of bacteria. One important additional observation of this work is although both complexes [Cu(sulfamethoxazole)2(H2O)4]⋅3H2O and [Cu(sulfamethoxazole)2]⋅H2O (Table 1) have the same ligand, the activity against E. coli and S. aureus was different due to the different stoichiometry and coordination environment.
With the aim to explain this behavior, a structural analysis was performed and permitted to propose the following hypothesis of mechanism of action.
It is known that sulfonamides act as Brönsted acids, according to the following reaction: Hsulfa = H+ + sulfa- (1)
The anionic form in reaction (1) is the antibacterial species but with low enough efficiency to penetrate across the lipoidal bacterial membrane [33].
One reason for the higher activity of these copper complexes may be due to the higher lipophilicity than the free sulfonamides. This is possible because Cu(II) blocks the polar residues of these ligands and, consequently, the penetration through the membrane should be improved. In addition, all this complexes coordinate through the heterocyclic N atom. This coordination maintains in a potential way the anionic form of sulfonamides. Also, if the Cu(II) is reduced to Cu(I) inside the cell as Ramadan proposes [34], the active form of sulfonamide would be liberated inside the cell.
The poor antibacterial activity of the other complexes could be caused by coordination through some groups, such as the aryl amine, which must be free in order to present antimicrobial activity.
Another result supporting this rational was obtained in the study of nickel-sulfonamide complexes [35] [Ni(sulfisoxazole)2(H2O)4]⋅2H2O presented equal MIC than the free ligand while the isostructural compound [Cu(sulfisoxazole)2(H2O)4]⋅2H2O was four-fold more active than sulfisoxazole for S. aureus and E. coli (Figure 3).
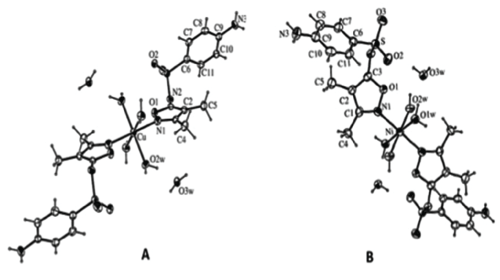
.
Figure 3: ORTEP view of the complexes [Cu(sulfisoxazole)2(H2O)4]⋅2H2O. (A) and [Ni(sulfisoxazole)2(H2O)4]⋅2H2O; (B) showing atom labeling and the 50% probability ellipsoids.
View Figure 3
This different activity was explained taking into account the impossibility that Ni(II) suffers bioreduction inside the cell affecting the liberation of sulfonamide.
This comparative study of copper and nickel complexes with sulfonamide is an example of the influence of the metal in the microbiological behavior. This kind of comparison is valid and important due to the fact that both complexes have the same stoichiometry and similar structure.
Fe(III) Compounds
Iron is an essential transition metal ion for the growth of pathogenic bacteria, which have different processes for iron acquisition. Due to the fact that iron can coordinate organic molecules presenting antimicrobial activity, it is possible to consider the process of iron acquisition as a possible strategy for the design of novel chemotherapeutic agents using this ion as a carrier of active drugs inside the cell.
In table 2 we can find new reports about iron complexes with antimicrobial activity that shows the current interest to continue studying these complexes.
Table 2: Iron complexes with antimicrobial activity.
View Table 2
The most active complexes reported in table 2 with triazol derivatives were active against Gram positive and Gram negative bacteria and the iron complexes with quinoxaline derivatives showed broader range of activity including M. tuberculosis.
Also, in the past, some interesting reports attracted the attention. Staphylococcus found in wound infections is very resistant to many antibiotics and is a great problem in hospitals. However it is very sensitive to the complexes [Fe(Me4phen)3]2+ and [Ru(Me4phen)2(acac)]+. These complexes are coordinatively saturated and so operated by physical interaction [13]. Another interesting compounds are the complexes formed by Fe(III) and the oxime 8-hydroxyquinoline. Albert showed that the free ligand and Fe(III) are both inactive against fungus and bacteria [13], but the complex with 1:1 molar ratio has important antifungal and antibacterial properties due to the fact that it is coordinatively unsaturated and charged and it bind too strongly to the membrane. Similar behavior presents the complex with 1:2 molar ratio. On the contrary, the complex with 3:1 metal:ligand ratio ([Fe(8-hydroxyquinoline)3]) is more lipophilic and it can penetrate inside the cell breaking down to the 2:1 and 1:1 complexes. The introduction of lipophobic groups into the oxime decreases the antibacterial activity.
Also, two novel iron complexes, [Fe(L-H)3], with 3- aminoquinoxaline-2-carbonitrile N1,N4-dioxide derivatives (L) as ligands (patent of invention PI0902923-0, 2009, Brazil) showed in vitro growth inhibitory activity on Mycobacterium tuberculosis H37Rv (ATCC 27294), together with very low unspecific cytotoxicity on eukaryotic cells (cultured murine cell line J774)] [38]. These complexes were synthesized in methanolic solution of Fe(III):ligands (1:2) and reacted under reflux many hours. The MIC values of both complexes were 0.78 μg mL-1 and for the free ligands were 3.9 for L8 and 6.2 for L6 (Table 2).
The general structure of these complexes is shown in figure 4.
Both complexes showed higher inhibitory effects than the standard second-line therapeutic drugs. Accordingly, these compounds could be useful as hospital desinfectants, as therapeutical agents for the treatment of skin infections produced by mycobacteria together with antiseptics and/or as drugs for the treatment of TB or other mycobacteriosis.
Zn(II) Compounds
Zn is an essential element present in a wide range of foods. Zinc salts have been tried in the treatment of the common cold with different results but there was no strong evidence to recommend their use [41].
Also, Zn supplementation reduced the incidence of pneumonia in children when compare with placebo, being an adjuvant to standard antimicrobial therapy [42].
In table 3 we can find new reports about zinc complexes with antimicrobial activity.
Table 3: Zinc complexes with antimicrobial activity.
View Table 3
Like the copper compounds, the heteroleptic zinc-complexes with ciprofloxacin were the most active against Gram positive and Gram negative bacteria.
In the research of information about mechanism of action, a good example to analyze is the case of [Zn4(xylH-4)2(H2O)2]⋅NaCl⋅3H2O (Figure 5). It was synthesized mixing methanolic solution of xylitol (0.2 mmol) and ZnCl2 (0.4 mmol) at 60°C and to the resulting solution adding methanolic NaOH until the formation of the complex.
This Zn-xylitol complex presented a good antibacterial activity against C. albicans and P. aeruginosa [47]. For example against P. aeruginosa, the MIC were 128 μgmL-1 and 64 μgmL-1 for the free xylitol and the zinc-xylitol complex respectively.
Xylitol is metabolized via the pentose pathway in humans, but it is unsuitable as an energy source for many microorganisms producing a xylitol-induced growth inhibition and disturbance in protein synthesis [57]. For this reason, it is used in the prophylaxis of several infections against S. pneumoniae [58], H. influenzae [59], M. catarrhalis [59], S. mutans [60], P. aeruginosa [59] and mucosal yeast infections in mammals, especially oral candidiasis which is the most common opportunistic infection in AIDS affecting up to 90% of the patients, among others [61].
The growth of C. albicans in the presence of [Zn4(xylH-4)2(H2O)2].NaCl⋅3H2O showed an 8 h delay at18h of incubation and a decrease of 30%, at 48 h,compared with the growth in presence of xylitol plus Zn+2. This behavior could be due to the higher lipophilicity value (P = 1.5) of the complex.
This study shows that the complex acts as a new species with an own behavior and its activity is not the result of a simple addition of the constituents.
Conclusions and Perspective
Numerous publications on the selected topic were found in the literature, revealing a great scientific interest in the field. Reviewed scientific information realizes many metal complexes having antimicrobial activity, which covers a broad range of microorganisms: Gram negative and positive bacteria, mycobacteria, yeast and fungi.
From the data analyzed it can be concluded that the strategy of metal coordination is a potent tool to get numerous and diverse new antimicrobial agents. Taking into account the MIC values and the spectrum of action, the most relevant compounds were the heteroleptic copper-complexes with CQ and zinc-complexes with ciprofloxacin against Gram negative and Gram positive bacteria. Moreover, the iron-complexes with tiazole and quinoxaline presented activity against M. tuberculosis, widening the spectrum of action.
Several Zn and Cu complexes presented antifungal activities standing out the [Zn(NMAPIMHMC)2]⋅2H2O and [Zn(TMPIMP)2]⋅2H2O for C. albicans.
Most part of the studied complexes is more active than the free ligands but there are scarce data on mechanisms of action. One might think that the greater lipophilicity of complexes favors the income through the microbial membranes and that the effect on the microbial targets is due to the action of the complexes as it, or metal and ligand as individual species where the complexes can act as a carrier of metals and ligands across the cell membrane. Moreover, few studies clarify on the synergistic action of metals and free ligands, information very valuable to make comparison with the activity of complexes, or include analysis of the selective action on microorganisms.
In consideration of the need for new antimicrobials to address the multi-resistance arose mainly in Gram negative bacteria, the metal complexes with antimicrobial activity are potential candidates to be used as an alternative or supplement to antibiotics. So further studies on selectivity of action, on antimicrobial activity with microorganisms exhibiting known antimicrobial biochemical mechanism of resistance, on the uptake mechanisms and identification of bacterial targets of these compounds are necessary and might be a new field of research.
In addition, many of the studied complexes showing a promising action on microorganisms that are difficult targets for antimicrobial drugs, are good candidates for further pharmaceutical studies. This fact determines the great potential of this strategy.
References
-
Gebeland H, Miller-Petrie M, Pant S, Gandra S, Levinson J, et al. (2015) The state of the world's antibiotics. CDDEP, Washington, DC.
-
Pavlović D, Fajdetić A, Mutak M (2010) Novel hybrids of 15-membered 8a- and 9a-azahomoerythromycin A ketolides and quinolones as potent antibacterials. Bioorg Med Chem 18: 8566-8582.
-
Center for Disease Dynamics, Economics & Policy (2015) State of the World’s Antibiotics, CDDEP, Washington, DC.
-
Lewis K (2013) Platforms for antibiotic discovery. Nat Rev Drug Discov 12: 371-387.
-
Yap PS, Krishnan T, Chan KG, Lim SH (2015) Antibacterial Mode of Action of Cinnamomum verum bark essential oil, alone and in combination with piperacillin, against a multi-drug-resistant Escherichia coli strain. J Microbiol Biotechnol 8:1299-1306.
-
Zazo H, Colino CI, Lanao JM (2016) Current applications of nanoparticles in infectious diseases. J Control Release 224: 86-102.
-
Gao W, Thamphiwatana S, Angsantikul P, Zhang L (2014) Nanoparticle approaches against bacterial infections. Wiley Interdiscip Rev Nanomed Nanobiotechnol 6: 532-547.
-
Lemire JA, Harrinson JJ, Turner RJ (2013) Antimicrobial activity of metals: mechanisms, molecular targets and applications. Nature Reviews Microbiology 11: 371-384.
-
Farrell N (Ed.) (1999) Uses of Inorganic Chemistry in Medicine. The Royal Society of Chemistry, Cambridge.
-
Clarke, MJ, PJ Sadler (1999) Metallopharmaceuticals II: Diagnosis and Therapy (Topics in biological inorganic chemistry) Vol. 2 Springer- Verlag, Berlin.
-
Gielen M, Tiekink ERT (2005) Metallotherapeutic Drugs and Metal-based Diagnostic Agents, The Use of Metals in Medicine Chichester. John Wiley & Sons.
-
Cotton FA, Wilkinson G, Murillo CA, Bochmann M (1999) Advanced Inorganic Chemistry, 6th Edition. Wiley-Interscience, New York.
-
Hughes N (1981) The Inorganic Chemistry of Biological Processes. Chichester: John Wiley & Sons, 300-315.
-
Sweetman SC, Blake PS, McGlashan JM, Neatherco GC (2006) Martindale: The Complete Drug Reference. 35th Edition, 1775.
-
Recio Despaigne AA, Da Costa FB, Piro OE, Castellano EE, Louro SRW, et al. (2012) Complexation of 2-acetylpyridine- and 2-benzoylpyridine-derived hydrazones to copper(II) as an effective strategy for antimicrobial activity improvement. Polyhedron 38: 285–290.
-
Martinez Medina JJ, Islas MS, Lopez Tevez LL, Ferrer EG, Okulik NB, et al. (2014) Copper(II) complexes with cyanoguanidine and o-phenanthroline: Theoretical studies, in vitro antimicrobial activity and alkaline phosphatase inhibitory effect. Journal of Molecular Structure 1058: 298-307.
-
Urquiza N, Islas MS, Dittler ML, Moyano MA, Manca SG, et al. (2013) Inhibition behavior on alkaline phosphatase activity, antibacterial and antioxidant activities of ternary methimazole-phenanthroline-copper (II) complex. Inorganica Chimica Acta 405: 243-251.
-
Liu H, Yang W, Zhou W, Xu Y, Xie J, et al. (2013) Crystal structures and antimicrobial activities of copper (II) complexes of fluorine-containing thioureido ligands. Inorganica Chimica Acta 405: 387-394.
-
Shakir M, Hanif S, Mohd Sherwani A, Mohammad O, Al-Resayes SA (2015) Pharmacologically significant complexes of Mn(II), Co(II), Ni(II), Cu(II) and Zn(II) of novel Schiff base ligand, (E)-N-(furan-2-yl methylene) quinolin-8-amine: Synthesis, spectral, XRD, SEM, antimicrobial, antioxidant and in vitro cytotoxic studies. Journal of Molecular Structure 1092: 143-159.
-
Kremer E, Facchin G, Estévez E, Alborés P, Baran EJ, et al. (2006) Copper complexes with heterocyclic sulfonamides: synthesis, spectroscopic characterization, microbiological and SOD-like activities: crystal structure of [Cu(sulfisoxazole)2(H2O)4] . 2H2O. J Inorg Biochem 100: 1167-1175.
-
Kiran S, Kumar Y, Puri P, Singh G (2012) Spectroscopic, thermal, and antimicrobial studies of Co(II), Ni(II), Cu(II), and Zn(II) complexes derived from bidentate ligands containing N and S donor atoms. Bioinorg Chem Appl, 729708.
-
Siddappa K, Mayana NS (2014) Synthesis, spectroscopic characterization, and biological evaluation studies of 5-Bromo-3-(((hydroxy-2-methylquinolin-7-yl)methylene)hydrazono)indolin-2-one and its metal (II) complexes. Bioinorg Chem Appl, 483282.
-
Raman N, Sakthivel A, Rajasekaran K (2007) Synthesis and spectral characterization of antifungal sensitive schiff base transition metal complexes. Mycobiology 35: 150-153.
-
Kharadi GJ (2011) Antitubercular and fluorescence studies of copper(II) complexes with quinolone family member, ciprofloxacin. Spectrochim Acta A Mol Biomol Spectrosc 79: 898-903.
-
Kharadi GJ (2014) Effect of substituent of terpyridines on the in vitro antioxidant, antitubercular, biocidal and fluorescence studies of copper (II) complexes with clioquinol. Spectrochimica Acta Part A: Molecular and Biomolecular 117: 662-668.
-
Abdel-Rahman LH, Abu-Dief AM, Ismael M, Mohamed MAA, Ali Hashem N (2016) Synthesis, structure elucidation, biological screening, molecular modeling and DNA binding of some Cu(II) chelates incorporating imines derived from amino acids. Journal of Molecular Structure 1103: 232-244.
-
Shaabani B, Khandar AA, Mobaiyen H, Ramazani N, Balula SS, et al. (2014) Novel pseudohalide-bridged Cu(II) complexes with a hydrazine ligand: evaluation of antimicrobial activity. Polyhedron 80: 166-172.
-
Ebrahimipour YS, Sheikhshoaie I, Crochet A, Khaleghi M, Fromm KM (2014) A new mixed-ligand copper (II) complex of (E)-N'-(2-hydroxybenzylidene) acetohydrazide: Synthesis, characterization, NLO behavior, DFT calculation and biological activities. Journal of Molecular Structure 1072: 267-276.
-
Pahonțu E, IlieșDS, Shova S, Paraschivescu C, Badea M, et al. (2015) Synthesis, characterization, crystal structure and antimicrobial activity of copper (II) complexes with the Schiff base derived from 2-Hydroxy-4-Methoxybenzaldehyde. Molecules 20: 5771-5792.
-
Ghaneian MT, Tabatabaee M, Ehrampoush MH, Jebali A, Hekmatimoghaddam S, et al. (2015) Synthesis of Ag (I) and Cu (I) complexes with 4-amino-5-methyl-2H-1,2,4-triazole-3(4H)-thioneligand as thiocarbohydrazide derivatives and their antimicrobial activity. Pharmaceutical Chemistry Journal 49: 210-212.
-
Borrás E, Alzuet G, Borrás J, Server-carrió J, Castiñeiras A, et al. (2000) Coordination chemistry of sulfamethizole: crystal structures of [Cu(sulfamethizolate)2(py)2(OH2)]⋅H2O, [M(sulfamethizolate)2(py)2(OH2)2] [M=Co and Ni] and {Cu(sulfamethizolate)2(dmf)2}∞. Polyhedron 19: 1859-1866.
-
Macías B, Villa MV, Fiz E, García I, Castiñeiras A, et al. (2002) Crystal structure of [Cu(N-quinolin-8-yl-p-toluenesulfonamidate)(2)]: study of its interaction with DNA and hydrogen peroxide. J Inorg Biochem 88: 101-107.
-
Foye WO, Lemke TL, Williams DA (1995) Principles of Medicinal Chemistry. 4th. ed. Baltimore:Williams & Wilkins ,709-713.
-
Ramadan AM (1997) Structural and biological aspects of copper (II) complexes with 2-methyl-3-amino-(3H)-quinazolin-4-one. J Inorg Biochem 65: 183-189.
-
Mondelli M, Bruné V, Borthagaray G, Ellena J, Nascimento OR, et al. (2008) New Ni (II)-sulfonamide complexes: Synthesis, structural characterization and antibacterial properties. X-ray diffraction of [Ni(sulfisoxazole)2(H2O)4]⋅2H2O and [Ni(sulfapyridine)2]. J Inorg Biochem 102: 285 -292.
-
Kumar G, Devi S, Johari R, Kumar D (2012) Synthesis, spectral characterization and antimicrobial evaluation of Schiff base Cr(III), Mn(III) and Fe(III) macrocyclic complexes. European Journal of Medicinal Chemistry 52: 269-274.
-
Kharadi GJ (2013) Antioxidant, tautomerism and antibacterial studies of Fe(III)-1,2,4-triazole based complexes. Spectrochim Acta A Mol Biomol Spectrosc 110: 311-316.
-
Tarallo MB, Urquiola C, Monge Vega A, Parajón Costa B, Ribeiro RR, et al. (2010) Design of novel iron compounds as potential therapeutic agents against tuberculosis. J Inorg Biochem104: 1164 - 1170.
-
Milenkovic' M, Cantoni G, Bacchi A, Spasojevic' V, Milenkovic' M, et al. (2014) Synthesis, characterization and antimicrobial activity of Pd(II) and Fe(III) complexes with ethyl (2E)-2-[2-(diphenylphosphino)benzylidene]hydrazinecarboxylate. Polyhedron 80: 47-52.
-
Elshaarawy RFM, Janiak C (2014) Toward new classes of potent antibiotics: Synthesis and antimicrobial activity of novel metallosaldach-imidazolium salts. European Journal of Medicinal Chemistry 75: 31-42.
-
Singh M, Das RR (2013) Zinc for the common cold. Cochrane Database of Systematic Reviews 6: CD001364.
-
Brooks WA, Yunus M, Santosham M, Wahed MA, Nahar K, et al. (2004) Zinc for severe pneumonia in very young children: double-blind placebo-controlled trial. Lancet 363: 1683-1688.
-
Demirezen N, Tarınç D, Polat D, Çeşme M, Gölcü A, et al. (2012) Synthesis of trimethoprim metal complexes: Spectral, electrochemical, thermal, DNA-binding and surface morphology studies. Spectrochim Acta A Mol Biomol Spectrosc 94: 243-255.
-
Tarushi A, Lafazanis K, Kljun J, Turel I, Pantazaki AA, et al. (2013) First- and second-generation quinolone antibacterial drugs interacting with zinc (II): Structure and biological perspectives. J Inorg Biochem 121: 53-65.
-
Shakir M, Hanif S, Asif Sherwani M, Mohammad O, Al-Resayes SI (2015) Pharmacologically significant complexes of Mn(II), Co(II), Ni(II), Cu(II) and Zn(II) of novel Schiff base ligand, (E)-N-(furan-2-yl methylene) quinolin-8-amine: Synthesis, spectral, XRD, SEM, antimicrobial, antioxidant and in vitro cytotoxic studies. Journal of Molecular Structure 1092: 143-159.
-
Kalinowska M, Piekut J, Bruss A, Follet C, Sienkiewicz-Gromiuk J, et al. (2014) Spectroscopic (FT-IR, FT-Raman, 1H, 13C NMR, UV/VIS), thermogravimetric and antimicrobial studies of Ca(II), Mn(II), Cu(II), Zn(II) and Cd(II) complexes of ferulic acid. Spectrochim Acta A Mol Biomol Spectrosc 122: 631-638.
-
Santi E, Facchin G, Faccio R, Barroso RP, Costa-Filho AJ, et al. (2016) Antimicrobial Evaluation of new metallic complexes with xylitol active against P. aeruginosa and C. albicans: MIC determination, post-agent effect and Zn-uptake. J Inorg Biochem 155: 67-75.
-
Yamgar RS, Nivid Y, Nalawade S, Mandewale M, Atram RG, et al. (2014) Novel zinc (II) complexes of heterocyclic ligands as antimicrobial agents: synthesis, characterisation, and antimicrobial studies. Bioinorg Chem Appl 2014: 276598.
-
Alaghaz, ANMA (2014) Synthesis, spectral characterization, molecular modeling and antimicrobial activity studies on 2-aminopyridine-cyclodiphosph(V)azane derivative and its homo-binuclear zinc (II) complexes. Journal of Molecular Structure1068: 27-42.
-
Raja Balan AMK, Ashok RFN, Vasanthi M, Prabu R, Paulraj A (2013) Synthesis, characterization, crystal structure and biological studies on nicotinanilide base nickel (II), copper (II) and zinc (II) complexes. Inorganica Chimica Acta 408:103-108.
-
Badiger DS, Hunoor RS, Patil BR, Vadavi RS, Mangannavar CV, et al. (2012) Synthesis, physico-chemical characterization and antimicrobial activities of 3-methoxysalicylaldehyde-2-aminobenzoylhydrazone and its transition metal complexes. Journal of Molecular Structure 1019:159-165.
-
Singh K, Kumar Y, Puri P, Kumar M, Sharma, C (2012) Cobalt, nickel, copper and zinc complexes with 1,3-diphenyl-1H-pyrazole-4- carboxaldehyde Schiff bases: Antimicrobial, spectroscopic, thermal and fluorescence studies. European Journal of Medicinal Chemistry 52: 313-321.
-
Kalanithi M, Kodimunthiri D, Rajarajan M, Tharmaraj, P (2011) Synthesis, characterization and biological activity of some new VO(IV), Co(II), Ni(II), Cu(II) and Zn(II) complexes of chromone based NNO Schiff base derived from 2-aminothiazole. Spectrochimica Acta - Part A: Molecular and Biomolecular Spectroscopy 82: 290-298.
-
El-Sherif AA, Eldebss TMA (2011) Synthesis, spectral characterization, solution equilibria, in vitro antibacterial and cytotoxic activities of Cu(II), Ni(II), Mn(II), Co(II) and Zn(II) complexes with Schiff base derived from 5-bromosalicylaldehyde and 2-aminomethylthiophene. Spectrochimica Acta - Part A: Molecular and Biomolecular Spectroscopy 79: 1803-1814.
-
Raman N, Pothiraj K, Baskaran T (2011) DNA interaction, antimicrobial, electrochemical and spectroscopic studies of metal(II) complexes with tridentate heterocyclic Schiff base derived from 2'-methylacetoacetanilide. Journal of Molecular Structure 1000: 135-144.
-
Patel M, Chhasatia M, Parmar P (2010) Antibacterial and DNA interaction studies of zinc(II) complexes with quinolone family member, ciprofloxacin. Eur J Med Chem 45: 439-446.
-
Tapiainen T, Kontiokari T, Sammalkivi L, Ikäheimo I, Koskela M, et al. (2001) Effect of xylitol on growth of Streptococcus pneumoniae in the presence of fructose and sorbitol. Antimicrob Agents Chemother 45: 166-169.
-
Froom J, Culpepper L, Jacobs M, DeMelker RA, Green LA, et al. (1997) Antimicrobials for acute otitis media? A review from the International Primary Care Network. BMJ 315: 98-102.
-
Ammons MCB, Ward LS, Fisher ST, Wolcott RD, James GA (2009) In vitro suceptibility of established biofilms composed of a clinical wound isolate of Pseudomonas aeruginosa treated with lactoferrin and xylitol. Int J Antimicrob Agents 33: 230-236.
-
Decker EM, Maier G, Axmannü D, Brecx M, von OhleC (2008) Effect of xylitol/chlorhexidine versus xylitol or chlorexidine as single rinses on initial biofilm formation of cariogenic streptococci. Quintessence Int 39: 17-22.
-
Vargas SL, Pearson J, Virkki M, Pepper T, Saunders F (2002) Use of polyols in combating yeast infection and polyol preparations for said use. US Patent 6414035 B1: 1-8.
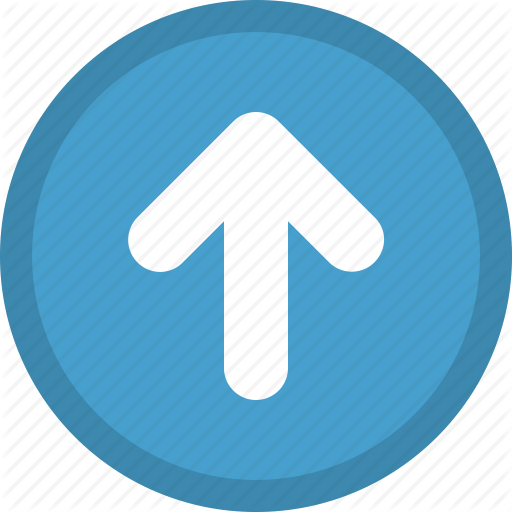