International Journal of Oral and Dental Health
The Challenge of Measuring Viscoelastic Properties of Human Whole Saliva to Fit Clinical Purpose
Diogo Löfgren C1*, Johansson D2, Bohlin L3, Sahlström A4 and Christersson C5
1Department of Materials Science and Technology and Department of Periodontology, Faculty of Odontology, Malmo University, 205 06 Malmo, Sweden
2Swedish Institute for Food and Biotechnology, SIK, Box 5401, 402 29 Goteborg and Department of Food Science, Swedish University of Agricultural Sciences, 750 07 Uppsala, Sweden
3Reologen i Lund AB, Lund, Sweden
4Reokonsa AB, 212 33 Malmo, Sweden
5Department of Materials Science and Technology, Malmo University, 205 06 Malmo, Sweden
*Corresponding author: Diogo Lofgren C, Department of Materials Science and Technology and Department of Periodontology, Faculty of Odontology, Malmo University, 205 06 Malmo, Sweden, E-mail: christina.diogo@mah.se
Int J Oral Dent Health, IJODH-1-017, (Volume 1, Issue 4), Research Article; ISSN: 2469-5734
Received: August 31, 2015 | Accepted: September 30, 2015 | Published: October 03, 2015
Citation: Diogo Lofgren C, Johansson D, Bohlin L, Sahlstrom A, Christersson C (2015) The Challenge of Measuring Viscoelastic Properties of Human Whole Saliva to Fit Clinical Purpose. Int J Oral Dent Health 1:017. 10.23937/2469-5734/1510017
Copyright: © 2015 Diogo LC, et al. This is an open-access article distributed under the terms of the Creative Commons Attribution License, which permits unrestricted use, distribution, and reproduction in any medium, provided the original author and source are credited.
Abstract
To understand the protective functions of saliva secreted from different glands in the masticatory process, it is of interest to study its viscoelastic properties. Characterization of saliva samples are not that easily performed in a clinical setting, since most of the experimental techniques and instruments available are developed for research purposes.
The aim of this study was to characterize how the viscoelastic properties of saliva can be measured and monitored using two laboratory instruments. Unstimulated whole saliva from 11 healthy volunteers was characterized using two instruments, an ARES-G2 rheometer and a Bohlin Oscillating Cup Rheometer. Measurements performed on unstimulated human whole saliva showed that the ARES rheometer will in linear viscoelastic conditions of the sample give absolute viscoelastic numbers of undisturbed saliva whilst the BOCR can be used to give an indication of gel strength, gel formation, and gel stability in viscoelastic samples being sheared in their non-linear viscoelastic region by introducing a Saliva Gel Strength Index, SGSI.
Both methods clearly illustrate the presence of viscoelastic properties in saliva.
Introduction
Saliva is a complex fluid composed of a wide variety of organic and inorganic constituents, which influence the biochemical and physiochemical properties of saliva, and thereby contribute to the numerous functions of saliva. Saliva has an important function in protecting oral soft tissues, teeth, and restorations from wear, demineralisation, dehydration and influence from external chemicals. In order to exercise this protective function, the saliva spreads out as a thin film over oral tissues, and if present, also over dental reconstructions [1-4].
Saliva is derived predominantly from three pairs of major glands (parotid, submandibular, and sublingual glands) that account for approximately 90% of the total fluid secretion. The minor salivary glands, whose ducts are open onto most areas of the oral mucosa except the area covering the dorsum of the tongue, the anterior part of the hard palate and gingivae, contribute to less than 10% of the total volume. The combination of secretions, entering the mouth at several locations, is termed whole saliva. In addition to the salivary secretions, whole saliva contains gingival crevicular fluid, oral microorganisms, host-derived cells, cellular constituents and dietary components [5].
It has been estimated that the total volume of saliva before and after swallowing averages about 1.1 and 0.8 ml, respectively [6]. Collins and Dawes [7] calculated that saliva if spread evenly throughout the mouth is present as a thin film between 70 and 100 μm thick.
A structural model of the salivary film has been developed and this model describes roughly 5 saliva layers including a precursor layer, a tightly bound protein layer, a layer of surface-anchored mucin brush, a liquid bulk layer and an outer proteinaceous layer at the interface with air [8,9]. This structure with multiple layers contributes to the entire spectrum of saliva's lubrication functionality [10].
The molecular composition of saliva has been shown to have multi-functional characteristics expressed by several families of salivary molecules, each comprising multiple members that are multifunctional and overlapping. Functional relationships are established upon complexing between molecules [11].
It is in particular salivary glycoproteins, such as mucins and proline-rich proteins, which have structural features that correlate to the protective function of masticatory lubrication. Mucins, of both high-molecular weight (MUC5B) and low-molecular weight (MUC7), are secreted from the submandibular-sublingual salivary glands while the proline-rich glycoproteins emanate from the parotid glands. These mucins form part of the acquired enamel pellicle and of the mucosal pellicle which forms a thin layer attached to the surface of the oral mucosa and they act as an important lubricant between opposing surfaces during mastication, swallowing and speaking [12,13].
The behaviour of saliva is based on both viscous and elastic properties and in order to understand the functions of saliva it is of interest to study these properties.
Currently, diagnostic methods are addressing quantity and content of saliva in bulk, and few qualitative tests of saliva, which can monitor dynamic changes either in bulk of saliva or of saliva as an adsorbed thin film, are at this date available to describe the protective functions of saliva. Since changes in the protective functions of saliva may occur that might influence oral health and the subject's perception of dry mouth, there is a need for functional tests in order to discern which individuals with oral dryness will require oral treatment. Salivary dysfunction has mainly been related to a decrease in salivary flow rate, but the molecular composition of saliva has gained more attention in understanding the complexity of the condition.
Studies have shown that there is no statistically significant or only a week correlation between the complaint of oral dryness and salivary flow rates [14-16]. This indicates that more complex physiological events than just the flow rate contribute to the symptom of oral dryness [17,18].
The above mentioned suggests that the rheological properties of saliva might be of greater importance for the perception of dry mouth than the quantity of saliva secreted alone.
The viscosity of whole saliva decreases upon increasing shear rate [19-21] and saliva can therefore be classified as a shear-thinning, non-Newtonian fluid with a high elastic component. Results from studies were rotational rheometers with cone-and-plate geometries were used to measure the shear thinning and the viscoelastic properties of saliva led to the assumption that saliva has gel-like properties. It has been shown that these measurements are partly erroneous since proteins in saliva adsorb to the air-liquid interface at the rim of rotational rheometers, resulting in formation of a solid-like elastic interface [22]. Hence, the rheological behaviour of saliva can be characterized as being shear thinning with a very high elastic component. Van der Reijden et al. [21] observed that the linear viscoelasticity and viscosity of saliva secreted from the various glands decreased in the following order: sublingual > palatal~whole saliva~submandibular > parotid. They suggested that the different rheological behaviour of saliva from each gland secretion could be due to an influence from mucin concentration, mucin conformation and/or the mucin type within the glandular saliva. The viscosity of submandibular and parotid saliva was shown to be hardly dependent on the shear rate opposite to sublingual saliva that showed a clear shear-thinning behaviour [21].
The shear rate independency of parotid saliva may be explained by the absence of high molecular weight mucins in this secretion, which gives it a viscosity slightly higher than that of water [23].
Furthermore, at similar viscosity submandibular saliva had a lower degree of elasticity compared to sublingual saliva. This might suggest that the structure that the sublingual mucins form is intrinsically more elastic than submandibular mucins.
The viscosity of saliva and its degree of shear thinning varies due to differences in measuring methods, saliva collection and handling, circadian rhythm, and individual variation [21,24-27], and this affects the feasibility of comparing results between studies.
Since saliva is a dilute viscoelastic polymer solution with very low shear modulus its viscoelasticity is therefore difficult to characterize experimentally. Davies and Stokes have developed an experimental technique, utilizing small gap distances, down to 5 μm, with parallel plate rheometry, which allows the use of small sample volumes. They demonstrated its applicability for high strains and strain rates as well as for viscoelasticity using small amplitude oscillatory shear (SAOS) [28]. The advantages of this SAOS technique as compared to previous measurements using oscillating capillary flow [21] and resonant oscillation [24,25,29] are a wide selection of frequencies and a well-defined strain.
Rheological characterization of saliva samples are not that easily performed in a clinical setting, since most of the experimental techniques often require large sized and expensive equipment developed for research purposes.
In order to explore the rheological properties of saliva, at the time when individuals have stated subjective complaints of oral dryness, the access to an instrument adapted to the clinical setting is a prerequisite. Another aspect is the stability of the sample. Rapid protein degradation occurs within 30 minutes after collection and the degradation starts during the collection procedure [30].
A surface loading rheometer, Bohlin Oscillating Cup Rheometer (Bohlin Reologi, Sjobo, Sweden) has been developed for measuring rheological properties of fluids. This technique is based on an oscillating system where the sample is initialized to perform free oscillations and where the damping caused by the sample is determined. This allows determination of viscosity and elasticity of low moduli fluids using small volume samples. In liquids with limited viscosity only a relatively thin film, which is in contact with the sample cup, participates in the oscillation [31]. This technique has been used for monitoring blood plasma coagulation [32-34].
In addition, the size of the instrument itself allows measurements in a clinical setting in contrast to a conventional controlled stress or strain rheometer.
The aim of the study was to characterize the viscoelastic properties of human whole saliva using two instruments, the ARES-G2 rheometer and the Bohlen Oscillating Cup Rheometer (BOCR).
Theory
Small amplitude oscillatory shear
The technique used with the ARES-G2 rheometer of small amplitude oscillatory shear with a narrow gap has been thoroughly described by Davies and Stokes [28]. In order to obtain meaningful measurements numerous gap errors have to be accounted for. The most prominent error, when using forced SAOS, is the unavoidable misalignment of parallel plates, which produces an underestimation in the measurements at gaps less than a few tenths of a millimetre. A typical gap error is 5-30 μm [26]. The gap error Δh is determined by measuring the viscosity ηm of a Newtonian liquid for decreasing set gap heights hs. A plot of hs/η vs. hs
Will give a straight line with slope 1/ηtrue and an intercept of Δh/ηtrue where ηtrue is the true viscosity of the Newtonian fluid. The gap error can then be used to give a corrected modulus through
where G refers to either G' or G".
Free amplitude oscillatory shear
The principle of the surface loading rheometer, like the BOCR, is to allow the sample, placed in a cup that is suspended by a torsion wire, to perform free oscillations and to measure the damping and frequency shifts of the oscillations caused by the sample. As the cylinder oscillates, the oscillation will penetrate into the fluid in the cylinder.
A hollow cylinder with an inner radius R and an inner height H is suspended by a torsion wire along its axis. The spring constant of this construction is Iωo2 wherein I, is the moment of inertia, and ωo the angular velocity, as the oscillation system with the empty cylinder performs free torsional oscillations. The free torsional oscillations are damped owing to the damping in the torsion wire and the other suspension means. The damping is defined by the logarithmic decrement Δ:
Wherein n is the number of periods of the oscillation and An is the amplitude of the oscillation in the nth period and A1 is the amplitude in the first period of oscillation. If the cylinder is filled with fluid and the oscillation system is caused to perform free oscillations, the damping of the oscillation will be greater than in the case of an empty cylinder. As the cylinder oscillates, the oscillation will penetrate into the fluid in the cylinder. The penetration depth δ is determined by the viscosity η, the density of the fluid ρ, and the angular velocity ω according to the equation:
By selecting a suitable oscillation frequency, a penetration depth delta δ of a low viscosity fluid can be obtained, which is much smaller than the radius R of the cylinder. In this case the fluid in the centre of the cylinder will be immovable during the torsional oscillations and the fluid between the cylinder wall and the immovable portion of the fluid will be subjected to shear. The shearing action promotes the damping of the oscillation. The damping caused by the shearing motion can be measured, and on the basis of the damping the viscosity of the fluid can be determined. For a viscous fluid the following relationship applies, provided that the penetration depth delta δ is much smaller than the radius R and the height H:
wherein Δ and Δ0 are the logarithmic decrement in oscillation with and without fluid in the cylinder, and k is a calibration constant. By measuring the damping and the relative frequency shift, the dynamic viscosity η' and the storage modulus G' can be determined for a slightly viscoelastic sample according to the following equations:
and
Wherein (-Δ ω/ωo) is the relative frequency shift [35].
An elastic response to free oscillation is a damping close to zero and a frequency shift corresponding to the density of the sample. This means that a viscoelastic material receives an extra frequency shift compared to a viscous sample, and that the elastic part of the saliva that oscillates in phase with the cup, is proportional to the strength of the saliva gel.
The relative frequency shift (ωo- ω)/ωo and the damping shift Δ-Δo constitutes the basic information we obtain concerning the sample behaviour in the oscillating cup.
For a viscoelastic liquid where the 'large cup' approximation (penetration depth << R) is valid we can calculate the dynamic viscosity and elasticity from equations (4) and (5) while the non-elastic liquid viscosity is given by equation (3). When there is no elasticity we have the relation
For the geometry and oscillation frequency used in the BOCR the large cup assumption is valid up to a viscosity of 10 cSt .For the saliva samples the situation is more complex since the Ares results points to a gel structure possibly with a yield stress that may vary among samples. It is obvious that we cannot use the 'large cup approximation' but need to use the basic information contained in the frequency and damping data.
We suggest that the saliva samples due to the gel property will give rise to an anomalous frequency shift in addition to that which would be the case if there was no elasticity, i.e.
(Δ-Δ0) and that the thickness of this layer of gel which is responsible for this increase in inertia will be proportional to the gel strength, which seems reasonable.
This results in the conclusion that in order to get a measure of the gel strength we should take the measured frequency shift and then subtract the frequency shift for a situation with no elasticity (Δ-Δ0). We then obtain something which is zero for no gel strength. We normalize arbitrarily by (Δ-Δ0) and finally obtain an empirical Saliva Gel Strength Index, SGSI.
Based on the SGSI assumption above, the BOCR can be used to give an indication of gel strength and gel formation in a viscoelastic sample, such as saliva at uncontrolled shearing conditions, corresponding to a saliva sample subjected to shearing in the mouth.
Materials and Methods
Collection of saliva
Unstimulated whole saliva of 11 healthy volunteers (5 women and 6 men, aged 34 ± 8 years) was collected. All individuals were instructed to refrain from smoking (including smokeless tobacco), eating, drinking or any oral hygiene measures for at least 1 h prior to the saliva collection procedure [36]. Saliva was collected during the hours between 10 am and 12 am or 2 pm and 4 pm.
The unstimulated whole saliva was collected when the participant was positioned in a relaxed position leaning slightly forward, such that saliva was allowed to pool in the mouth. The saliva first expectorated was discarded. The donor then expectorated saliva into a test tube for 5-10 min until 2 ml of saliva was collected.
The rheological properties of saliva were immediately measured within 2 min after collection.
The sample collected from the donor was calculated to enough volume to be used for both techniques.
Rheological measurements of unstimulated whole saliva
The small amplitude oscillatory shear rheology of unstimulated whole saliva was characterized by using an ARES-G2 rheometer (TA Instruments, New Castle, DE, USA) equipped with a parallel plate measuring system (40 mm diameter plates). The gap error was first determined to be Δh = 2.8 μm using a Newtonian calibration oil with a viscosity of 318.7 mPa s at 37°C.
220 μl of the saliva was pipetted to the centre of the bottom plate. The upper plate was lowered to a gap of 100 μm and an oscillatory time sweep with frequency 0.1 Hz was started immediately. Data was recorded for 20 minutes. The sample was covered with a thin layer of a low viscosity paraffin oil to avoid drying of the sample. The measurements were performed in the linear viscoelastic region using an applied strain of 5%. All measurements were performed at 37°C.
The free oscillatory rheology of unstimulated whole saliva was characterized using a Bohlin Oscillating Cup Rheometer (BOCR) (Bohlin Reologi, Sjobo, Sweden) (Figure 1). Prior to measurements the instrument was calibrated with 1.0 ml of distilled water. The water was removed from the sample cell and replaced by 1.0 ml whole saliva for recording during 23 min. The sample cell was used without a fixed bob during the measurements. All measurements were carried out in room temperature (21.4°C-22.6°C).

.
Figure 1: The Bohlin Oscillating Cup Rheometer (BOCR).
1. Oscillator actuator, sensor and suspension assembly.
2. Base mount
3. Sample cell.
4. Fixed Central cylinder for best thermal control.
5. Thermal jacket
6. Cover plate.
View Figure 1
Results and Discussion
Viscoelastic data
As can be seen from figure 2 the storage modulus, G', of most of the saliva samples during linear shear increase as a function of time and then stabilises after about 1200 s. Further, table 1, displaying the storage modulus, G', and the phase angle, δ, of the samples after 1200 s shows that most of the saliva samples show a high degree of viscoelasticity and a moderate level of elasticity, with all samples but four, S7, S8, S9 and S11, having phase angles < 30° and storage modulus between 0,15 and 2.1 Pa when subjected to linear behaviour, i.e. at rest. The elastic behaviour of the samples may be due to the adsorption of proteins at the air-liquid interface. Therefore, the increase in elasticity as a function of time demonstrates the formation of an entanglement structure in saliva. It is clearly seen that the degree of elasticity differs between the saliva samples and this can be explained by lower or higher average molecular weight of the structure forming proteins, due to compositional differences of the saliva, or the concentration of the same in saliva. An increase in the average molecular weight as well as protein concentration would be expected to result in a higher storage modulus. The linear measurement results obtained hence indicate that samples S3, S6, S4 and S2, with G' values between 1.2 and 2.1 Pa and δ values below 30° have the highest degree of structure. The variation in G' value as a function of time as well as the more viscous behaviour for sample S11 can be an example of a very dilute saliva sample with too low degree of elasticity in relation to the sensitivity of the rheometer. The solid-like surface film developed in saliva at rest, discussed above, has been shown to be disrupted at very low strains [22].
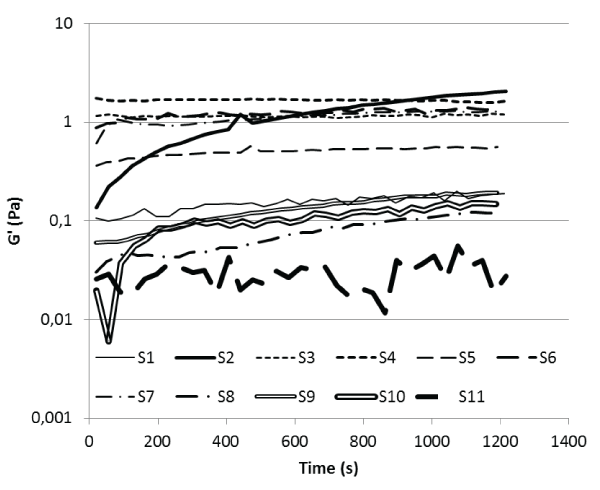
.
Figure 2: Ares viscoelastic data.
Saliva storage modulus (G') as a function of time at T = 37°C
Each participant denoted as S for subject.
View Figure 2
Table 1: Storage modulus and phase angle values of the samples studied after 1200s at 37°C.
View Table 1
It can be seen from figure 3 that the Gel strength index, SGSI, of most of the saliva samples show linear elastic values during the time of the measurement. The reason for this linear behaviour compared to the increase in elasticity for the ARES measured saliva samples can be explained by the measurement parameters. The ARES measurements are performed in the linear viscoelastic region of the saliva.
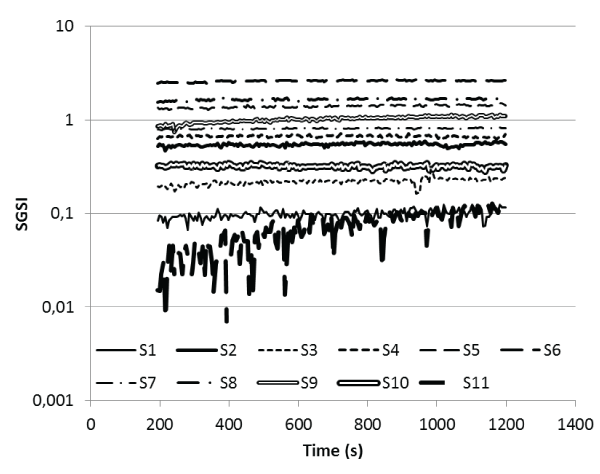
.
Figures 3: BOCR viscoelastic data.
SGSI = η'/(η-1) plotted as a function of time at T = 22°C
Each participant denoted as S for subject.
View Figures 3
This means that we study the saliva samples without any significant impact on their structure which influence the rheological properties. The BOCR measurements are performed in uncontrolled and most probably non-linear viscoelastic region of the saliva samples, preventing the formation of solid-like surface films. As for the ARES measurements it is clearly seen that the degree of elasticity differs between the saliva samples and this may again explained by the concentration and composition, with the resulting differences in average molecular weight, of the structure forming proteins as well as their resistance to breakdown during shear in the mouth. The viscosity of the saliva samples in the BOCR, ranged between 0.69-3.56 mPa s and with an average of 1.77 mPa s this is in line with what has previously been measured.
Salivary mucins have been reported to comprise up to 26% of the total protein (1-2 mg/ml) concentration in saliva [37]. Of these mucins, MUC5B has by far the highest molecular weight, Mw reaching up to 2-40 MDa and the concentration of the same has been reported as 233 μg/ml [38]. Based on this it can be expected that MUC5B dominates the behaviour of saliva. On the other hand, it is simplistic to consider saliva as a solution of MUC5B since it has been shown that concentrated solutions of this mucin do not fully replicate the gel-like properties of human whole saliva. Furthermore, the aggregation of other mucins, such as MUC7, and the presence of various lubricative and surface active molecules also play a role in the behaviour of saliva [39].
The conformation of mucin depends on factors such as ionic strength and pH [40]. Bicarbonate is a vital component of the pH buffering system. The concentration of bicarbonate increases markedly with the flow rate and it is therefore only really effective at high salivary flow rates. The bicarbonate ion reduces the amount of free calcium and calcium bound to mucins, and this may cause mucins to lose the ability to crosslink resulting in a more relaxed structural arrangement and decreased viscosity [41].
At lower pH, mucins are reported to form gel phases due to hydrophobic interactions resulting in an increased tendency for aggregation [40,42].
It could be argued that the difference in degree of elasticity between samples partly is a result of individual variations in pH. Since unstimulated saliva was characterized in the present study and the fact that the bicarbonate concentration, which affects the conformation of mucins, increases with flow rate the alleged variations in pH might be a minor contributing factor to the differences seen between samples.
Saliva has a unique combination of rheological properties, namely low viscosity, shear thinning and high elasticity which most likely are responsible for the elastohydrodynamic (full-film) lubricating properties. Since saliva has a high stress ratio [27], a relatively thin salivary film is able to keep opposing surfaces apart. The low viscosity combined with shear thinning results in low friction. An alteration in these properties may lead to an impairment in the lubricating ability of the oral mucosa and other oral tissues and result in abrasion, erosion, tooth decay, as well as it may affect the subjective sensation of dryness.
Since the lubrication properties of saliva is a function of the rheological characteristics and the surface associated components as well as the bulk components and their interplay [25] a multitechnological approach is needed in order to explore and characterize factors that influence the protective functions of saliva.
Conclusions
The ARES rheometer is an absolute instrument and will in linear viscoelastic conditions of the sample give absolute viscoelastic numbers of undisturbed saliva.
The BOCR can be used to give an indication of gel strength and structure resistance to breakdown during shear in viscoelastic samples being sheared in their non-linear viscoelastic region through assuming a Saliva Gel Strength Index, SGSI.
It is suggested that the BOCR can be used to distinguish between saliva samples with high and low degree of viscoelasticity respectively. This assumption is also supported by the fact that shearing of the saliva in the mouth is rather a non-linear than a linear shearing process.
Considering that time will affect the dynamic molecular rearrangements in the saliva sample and thereby the shearing process, the BOCR will be a clinically suitable method to user chair side for measuring viscoelastic behaviour of saliva. Further studies are undertaken to analyse samples from patients with dry mouth symptoms, to validate the accuracy of the BOCR measurements for diagnostic purpose as well as to characterize the protective functions of saliva with a multitechnological approach
References
-
Baier RE, Glantz PO (1978) Characterization of oral in vivo films formed on different types of solid surfaces. Acta Odontol Scand 36: 289-301.
-
Meckel AH (1965) The formation and properties of organic films on teeth. Arch Oral Biol 10: 585-598.
-
Rolla G (1977) Formation of dental integuments-some basic chemical considerations. Swed Dent J 1: 241-251.
-
Sonju T, Glantz PO (1975) Chemical composition of salivary integuments formed in vivo on solids with some established surface characteristics. Arch Oral Biol 20: 687-691.
-
Leone CW, Oppenheim FG (2001) Physical and chemical aspects of saliva as indicators of risk for dental caries in humans. J Dent Educ 65: 1054-1062.
-
Lagerlof F, Dawes C (1984) The volume of saliva in the mouth before and after swallowing. J Dent Res 63: 618-621.
-
Collins LM, Dawes C (1987) The surface area of the adult human mouth and thickness of the salivary film covering the teeth and oral mucosa. J Dent Res 66: 1300-1302.
-
Veeregowda DH, Busscher HJ, Vissink A, Jager DJ, Sharma PK, et al. (2012) Role of structure and glycosylation of adsorbed protein films in biolubrication. PLoS One 7: e42600.
-
Lundin M, Sandberg T, Caldwell KD, Blomberg E (2009) Comparison of the adsorption kinetics and surface arrangement of 'as received' and purified bovine submaxillary gland mucin (BSM) on hydrophilic surfaces. J Colloid Interface Sci 336: 30-39.
-
Yakubov GE (2014) Lubrication. Monogr Oral Sci 24: 71-87.
-
Levine MJ (1993) Salivary macromolecules. A structure/function synopsis. Ann N Y Acad Sci 694: 11-16.
-
Siqueira WL, Zhang W, Helmerhorst EJ, Gygi SP, Oppenheim FG (2007) Identification of protein components in in vivo human acquired enamel pellicle using LC-ESI-MS/MS. J Proteome Res 6: 2152-2160.
-
Gibbins HL, Proctor GB, Yakubov GE, Wilson S, Carpenter GH (2014) Concentration of salivary protective proteins within the bound oral mucosal pellicle. Oral Dis 20: 707-713.
-
Fox PC, Busch KA, Baum BJ (1987) Subjective reports of xerostomia and objective measures of salivary gland performance. J Am Dent Assoc 115: 581-584.
-
Sreebny LM, Valdini A (1988) Xerostomia. Part I: Relationship to other oral symptoms and salivary gland hypofunction. Oral Surg Oral Med Oral Pathol 66: 451-458.
-
Hay EM, Thomas E, Pal B, Hajeer A, Chambers H, et al. (1998) Weak association between subjective symptoms or and objective testing for dry eyes and dry mouth: results from a population based study. Ann Rheum Dis 57: 20-24.
-
Fox PC, van der Ven PF, Sonies BC, Weiffenbach JM, Baum BJ (1985) Xerostomia: evaluation of a symptom with increasing significance. J Am Dent Assoc 110: 519-525.
-
Lofgren CD, Isberg PE, Christersson C (2010) Screening for oral dryness in relation to salivary flow rate addresses the need for functional tests of saliva. Oral Health Prev Dent 8: 243-252.
-
Schwartz WH (1987) The rheology of saliva. J Dent Res 66: 660-664.
-
Vissink A, Waterman HA, s-Gravenmade EJ, Panders AK, Vermey A (1984) Rheological properties of saliva substitutes containing mucin, carboxymethylcellulose or polyethylenoxide. J Oral Pathol 13: 22-28.
-
van der Reijden WA, Veerman EC, Amerongen AV (1993) Shear rate dependent viscoelastic behavior of human glandular salivas. Biorheology 30: 141-152.
-
Waterman HA, Blom C, Holterman HJ, 's-Gravenmade EJ, Mellema J (1988) Rheological properties of human saliva. Arch Oral Biol 33: 589-596.
-
Veerman EC, Valentijn-Benz M, Nieuw Amerongen AV (1989) Viscosity of human salivary mucins: effect of pH and ionic strength and role of sialic acid. J Biol Buccale 17: 297-306.
-
Mellema J, Holterman HJ, Waterman HA, Blom C, Gravenmade EJ (1992) Rheological aspects of mucin-containing solutions and saliva substitutes. Biorheology 29: 231-249.
-
Christersson CE, Lindh L, Arnebrant T (2000) Film-forming properties and viscosities of saliva substitutes and human whole saliva. Eur J Oral Sci 108: 418-425.
-
Rantonen PJ, Meurman JH (1998) Viscosity of whole saliva. Acta Odontol Scand 56: 210-214.
-
Stokes JR, Davies GA (2007) Viscoelasticity of human whole saliva collected after acid and mechanical stimulation. Biorheology 44: 141-160.
-
Davies GA, Stokes JR (2008) Thin film and high shear rheology of complex fluids. J Non-Newtonian Fluid Mech 148: 73-87.
-
Van der Reijden WA, Veerman EC, Nieuw Amerongen AV (1994) Rheological properties of commercially available polysaccharides with potential use in saliva substitutes. Biorheology 31: 631-642.
-
Esser D, Alvarez-Llamas G, de Vries MP, Weening D, Vonk RJ, et al. (2008) Sample Stability and Protein Composition of Saliva: Implications for Its Use as a Diagnostic Fluid. Biomark Insights 3: 25-27.
-
Bohlin L (1994) Method of measuring rheological properties and rheometer for carrying out the method. PCT (Patent Cooperation Treaty) publication number WO94/08222.1994.
-
Ranby M, Gustafsson KM, Lindahl TL (1999) Laboratory diagnosis of thrombophilia by endothelial-cell-modulated coagulation. Blood Coagul Fibrinolysis 10: 173-179.
-
Ranby M, Ramstrom S, Svensson PO, Lindahl TL (2003) Clotting time by free oscillation rheometry and visual inspection and a viscoelastic description of the clotting phenomenon. Scand J Clin Lab Invest 63: 397-406.
-
Hansson KM, Tengvall P, Lundstrom I, Ranby M, Lindahl TL (2002) Surface plasmon resonance and free oscillation rheometry in combination: a useful approach for studies on haemostasis and interactions between whole blood and artificial surfaces. Biosens Bioelectron 17: 747-759.
-
Bohlin L (1996) A surface loading rheometer. In: Proc. Nordic Rheology Conference, Stavanger, Norway.
-
Navazesh M, Kumar SK; University of Southern California School of Dentistry (2008) Measuring salivary flow: challenges and opportunities. J Am Dent Assoc 139: 35-40.
-
Thornton DJ, Khan N, Mehrotra R, Howard M, Veerman E, et al. (1999) Salivary mucin MG1 is comprised almost entirely of different glycosylated forms of the MUC5B gene product. Glycobiology 9: 293-302.
-
Rayment SA, Liu B, Offner GD, Oppenheim FG, Troxler RF (2000) Immunoquantification of human salivary mucins MG1 and MG2 in stimulated whole saliva: factors influencing mucin levels. J Dent Res 79: 1765-1772.
-
Raynal BD, Hardingham TE, Thornton DJ, Sheehan JK (2002) Concentrated solutions of salivary MUC5B mucin do not replicate the gel-forming properties of saliva. Biochem J 362: 289-296.
-
Bansil R, Turner BS (2006) Mucin structure, aggregation, physiological functions and biomedical applications. Curr Opin Colloid Interface Sci 11: 164-170.
-
Chen EY, Yang N, Quinton PM, Chin WC (2010) A new role for bicarbonate in mucus formation. Am J Physiol Lung Cell Mol Physiol 299: 542-549.
-
Svensson O, Arnebrant T (2010) Mucin layers and multilayers-Physicochemical properties and applications. Curr Opin Colloid Interface Sci 15: 395-405.
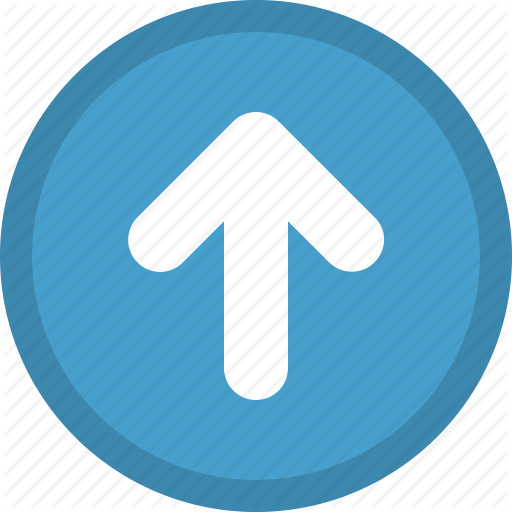