International Journal of Sports and Exercise Medicine
A Comprehensive Kinematic Analysis during a 15 Km Submaximal Training Run
Joshua P. Bailey* John A. Mercer and Janet S. Dufek
Kinesiology and Nutrition Sciences, University of Nevada, Las Vegas, USA
*Corresponding author: Joshua P. Bailey, Kinesiology and Nutrition Sciences, University of Nevada, Las Vegas, NV, 4505 S Maryland Parkway, USA, Tel: 702-406-7470, E-mail: bailey69@unlv.nevada.edu
Int J Sports Exerc Med, IJSEM-2-039, (Volume 2, Issue 2), Original Article; ISSN: 2469-5718
Received: December 04, 2015 | Accepted: February 25, 2016 | Published: April 02, 2016
Citation: Bailey JP, Mercer JA, Dufek JS (2016) A Comprehensive Kinematic Analysis during a 15 Km Submaximal Training Run. Int J Sports Exerc Med 2:039. 10.23937/2469-5718/1510039
Copyright: © 2016 Bailey JP, et al. This is an open-access article distributed under the terms of the Creative Commons Attribution License, which permits unrestricted use, distribution, and reproduction in any medium, provided the original author and source are credited.
Abstract
Purpose: The purpose of the study was to evaluate kinematics during a 15 km submaximal endurance training run.
Methods: Fifteen participants (eight male, seven female; 30.5 ± 8.4 years; 71.8 ± 11 kg; 1.73 ± 0.07 m) currently training for an endurance race completed a 15 km training run (1:24:34 ± 0:10:20 finish times) on an outdoor course. Sagittal plane two-dimensional kinematic data were captured and analyzed for a single stance phase at 0.5 km intervals. All dependent variables (lap times, step length, step frequency, stance time, and joint angles (knee, ankle & hip) at contact and during stance) were calculated every half km and averaged over three km segments.
Results: Lap time (p < 0.001) and stance time (p < 0.001) each increased across the duration of the run. Step length decreased (p = 0.020) but there was no change in step frequency (p = 0.087) across time. Knee flexion at foot contact decreased across time (p = 0.041), with no changes in hip (p = 0.916) or ankle (p = 0.591) angles at foot contact. Torso inclination increased across laps (p = 0.001). During stance phase, decreased peak knee flexion (p = 0.041) was the only lower extremity joint angle altered during the run.
Conclusion: Runners adjust lower and upper extremity body positions, as well as running gait parameter variables, in response to slower running velocities during the later stages of a submaximal endurance run.
Keywords
Recreational runners, Running mechanics, Overground running, Fatigue, Exhaustion
Introduction
Recent studies have investigated biomechanics of running during competitive road races in an attempt to record endurance runners in their natural environment, due to the ability to measure numerous runners in a short period of time [1-4]. A key focus area for running races are the foot strike pattern differences among foot wear conditions [5], running speed measured as finishing time [3,4] and changes related to fatigue during later stages of the race [1,2,6]. Identification utilizing two-dimensional video analysis has shown that under all conditions, majority of endurance runners possess a rear-foot striking pattern. Fewer studies have expanded the kinematic analysis beyond foot strike patterns in an effort to identify other variables (e.g., joint angles and foot contact position relative to the center of mass) related to injury prevention and performance [1,2,6]. The motivation behind the study of endurance runners in their natural environment was to understand how they might alter running mechanics throughout an endurance run.
A key component within race studies is the possible influence of the competitive nature on the kinematic changes reported. The competitive nature may cause the runner to push through pain and possible fatigue in order to meet a performance goal. Additionally, any evaluations and changes were measured at a limited number, one to three, of data acquisition locations. This limited number of analysis points may ignore numerous adjustments runners make throughout the race in response to external or internal stimuli.During the training program for these endurance races, runners perform numerous runs without the competitive influence. It has been estimated that upwards of 60% of endurance running injuries are associated with training errors [7].
There is a gap in the literature that it is not known how (if at all) kinematics change during the course of a long endurance run lacking the competitive nature of a race. Given the importance of training endurance runs on the etiology of endurance running overuse injuries, the current study attempts to evaluate running kinematics during a training endurance run. It was hypothesized that runners may adjust running mechanics during a non-competitive training run, especially gait parameters due to the runners' ability to self-optimize. It was further hypothesized that these mechanical changes would be adjusted differently at different phases of the run. Therefore, the current research purpose was to evaluate kinematics at half km intervals during a 15 km submaximal training run outdoors.
Methods
Participants
A convenience sample was taken from local running groups and students attending the local university. Fifteen runners (eight male, seven female; 30.5 ± 8.4 years; 71.8 ± 11 kg; 1.73 ± 0.07 m) currently training for any endurance race (23.8 ± 4.6 miles/week; range 20-35 miles/week) gave written consent to participate in the university-approved study. Inclusion criteria for participation in the study were an age range of 18-45 years and weekly running mileage of at least 20 miles. Additionally, each runner had to be able to complete the 15 km distance run in 2 hours or less. All participants were generally healthy and free from any lower extremity injury, which would have side-lined them from running for at least two weeks within the previous six months.
Instrumentation
The data collection and 15 km run was conducted entirely outdoors. Although the run course included a mixture of terrain, the data collection section of the course had subjects running on concrete with analysis occurring in the same location every lap. The analysis area was located within the last 15 m of a section 150 m straight path of concrete walkway, which was oriented to reduce possible changes in gait parameters and lower extremity kinematic adjustments as a result to changes in the path. An attempt was made to supply ample time for the runners to achieve a steady running gait pattern during the straight path approaching the analysis area.
A Basler GigE Scout high-speed camera (model scA640-120gm; Exton, PA) with a Pentax CCTV 8-48 mm lens (Hoya Corporation, Tokyo, Japan) was placed nine meters perpendicular to a designated path (1.02 m wide) that the runner would follow capturing sagittal kinematics. Data were captured via MaxTraq two-dimensional video acquisition and digitization software (Innovision Systems Inc. 2.2.2.5; Columbiaville, MI) at 120 Hz with a resolution of 659 × 494 pixels.
Six high contrast markers were placed on landmarks of the lower extremity and upper torso for digitization purposes: 1) lateral aspect of neck inferior to ear (Neck), 2) iliac crest of pelvis (Torso), 3) greater trochanter (Grtro), 4) lateral aspect of the knee joint center (Knee), 5) lateral malleolus (Ankle), and 6) lateral aspect of the shoe corresponding to the lateral aspect of the fifth metatarsal (Foot). Markers were attached using athletic cover-roll stretch tape (BSN medical, Inc., Charlotte, NC). For areas that were prone to excess sweating, Tuf-Skin (Cramer Products, Inc., Gardner, Kansas) was used to increase adhesion of the markers.
Procedure
Following marker placement, participants performed a self-directed warm-up lasting at least five minutes, but no more than ten minutes. Following warm-up, participants were informed of the half km marked running path around the campus of the local university, which combined both concrete and asphalt surfaces. Three participants (P10, P11 and P12) ran a course of 0.54 km laps due to campus construction interference with the original course. To complete the 15 km training run, participants were informed that they would need to complete 30 laps (by themselves), with the same goal as if they were going out for a normal training 15 km run. It was stressed to each participant that the run was to be completed as if they were running a normal run in their weekly training program at their self-selected speed, finishing in less than 2 hours. Participants were informed that they would be videotaped every half kilometer and encouraged to run naturally using preferred kinematics through the video area. All participants successfully completed the 15 km course in the time required (< 2 hrs).
Data Analysis
The six markers were digitized in two phases. The first was to identify a single stride as the runner passed through the test area of the course. The second was to identify a single stance phase in which the stance phase occurred for the right leg. Visual identification of the stance phase was determined by denoting the frame in which any portion of the right foot made initial contact with the running surface to the frame in which the foot lost contact (toe-off) with the running surface. From the initial contact and toe-off frame acquisition, running parameters were extracted: step frequency, step length, and stance phase (Stance). Step frequency and step length data were extracted from the digitized consecutive left-right and right-left initial contacts. Ankle, knee and hip joint angles were extracted from the digitized markers, in addition to the torso inclination.
A total of 30-stance phase periods over the course of the 15 km run were recorded (i.e., one per lap). Stance phases were grouped together in six lap (approximately three km) combinations, creating five circuits (Circuit_1, Circuit_2, Circuit_3, Circuit_4, Circuit_5). Collapsing across six laps provided the ability to identify possible changes during the beginning and end of the run, with further identification of possible adjustments at intermediate points of the run. The approximate three km distance may represent splits used during endurance runs to monitor training.
All dependent variables (DVs), including Lap time, gait parameters (step frequency, step length and Stance), angle measurements at initial contact (Torso inclination, Hip angle, Knee angle, and Ankle angle) and the peak flexion during stance period (Hip peak flexion angle, Knee peak flexion angle and Ankle peak flexion angle) were analyzed across laps. Each circuit consisted of averaging each parameter across six consecutive laps. Additionally, the greater trochanter vertical displacement (greater trochanter vertical excursion) was calculated as the difference in vertical position of the greater trochanter at foot contact and the lowest vertical position of the greater trochanter during the stance phase. The greater trochanter vertical displacement was used to represent the center of mass vertical excursion during the stance phase.
All DVs were extracted from MaxTraq using a customized Matlab code. Joint angles during stance phase were smoothed using a Low-Pass 4th Order Butterworth Zero-lag filter.
Statistical Analysis
Descriptive statistics for each dependent variable were reported as a six trial mean ± standard deviation for each circuit. Each dependent variable (Lap time, step length, step frequency, Stance, angles at initial contact, peak flexion angle during stance, and greater trochanter vertical excursion) using SPSS Statistics software (IBM; Armonk, NY) was compared across circuits using one-way repeated measure analyses of variance (ANOVAs; α = 0.05). The assumption of sphericity was tested using Mauchly's test, with Huynh-Feldt corrections made for violations of p < 0.05. When appropriate, Sidak post-hoc tests were run to determine differences among Circuits.
Results
Descriptive statistics (mean ± standard deviation) for Lap times and step characteristics are reported in Table 1. Lap time was different across circuits (F(2.790, 39.065) = 15.829, p < 0.001, η2 = 0.531). Participants ran the fastest in the beginning of the run with lap times for Circuit _1 faster than each subsequent Circuit (p < 0.05). Circuit 2 was slower than Circuit_1 (p = 0.002), but faster than both Circuit_3 (p = 0.010) and Circuit_4 (p = 0.012). Stance was different across circuits (F(2.427, 33.983) = 8.877, p < 0.001, η2 = 0.388). Stance was shorter during Circuit_1 compared to Circuit_2 (p = 0.011), Circuit_3 (p = 0.030) and Circuit_4 (p = 0.007). Step length was different across circuits (F(1.220, 17.077) = 5.998, p = 0.021, η2 = 0.300) with a gradual decrease in step length from Circuit_1 to Circuit_4. Step frequency was not different across circuits (p = 0.279).
Table 1: Descriptive statistics (mean ± standard deviation) for Lap times and step characteristics.
View Table 1
Descriptive statistics (mean ± standard deviation) for angles at initial contact are reported in Table 2. Knee angle at initial contact was different across circuits (F(4, 56) = 2.674, p = 0.041, η2 = 0.160). Participants ran with a more flexed knee during Circuit_1 than Circuit_5 (p = 0.048). Both Hip angle at initial contact (p = 0.916) and Ankle angle at initial contact (p = 0.591) were not different across circuits. Torso inclination was different across circuits (p = 0.001).
Table 2: Descriptive statistics (mean ± standard deviation) for angles at initial contact.
View Table 2
Knee peak flexion angle was different across circuits (F(4, 56) = 3.304, p = 0.017, η2 = 0.191). Participants ran with less peak knee flexion during Circuit_2 than Circuit_5 (p = 0.041). There were no differences identified for Ankle peak flexion angle (p = 0951), Hip peak flexion angle (p = 0.591), or greater trochanter vertical displacement (p = 0.693) among circuits during the stance period Table 3.
Table 3: Descriptive statistics (mean ± standard deviation) for peak joint flexion and greater trochanter vertical excursion during stance.
View Table 3
Discussion
The purpose of this study was to evaluate possible running kinematic changes during a 15 km submaximal training run. The runners chosen for the study were recreational runners, non elite, currently training for an endurance race because they could complete the length of the run and have been reported to have an increased rate of overuse injury related to training errors [8-10]. Furthermore, the kinematic variables of interest were analyzed numerous times in an attempt to capture continual adjustments made throughout the run, which differed from the majority of race studies which analyzed runners only a few times in an effort to see differences between the early and later stages of the race.
In response to the original hypothesis, the majority of significant differences measured throughout the run were gait parameters. Lap times were shorter during the beginning of the run followed by a decrease until the final Circuit, indicating a slowing running speed following the first three km. Runners during the race studies have shown a similar pacing effect with the fastest times coming during the initial stages of the run, only to reduce their running speed throughout the run in an effort to conserve for a surge at the end of the run [11]. The runners in the research study, although it was supposed to represent a normal run, may have felt pressure to perform and started running too fast, quickly realizing the need to reduce speed. The course that was designed for the study created a half km loop, which may have reduced the motivation throughout the run, aiding in the reduction in running speed during the middle stages of the run. The non-significant difference in Lap times for the final three Circuits indicates that the runners were able to maintain a comfortable running speed following the initial six kilometers. The measured running speed changes throughout the run were affected by the grouping of the laps into Circuits and it is important to note that a different grouping pattern may indicate differing results Figure 1.
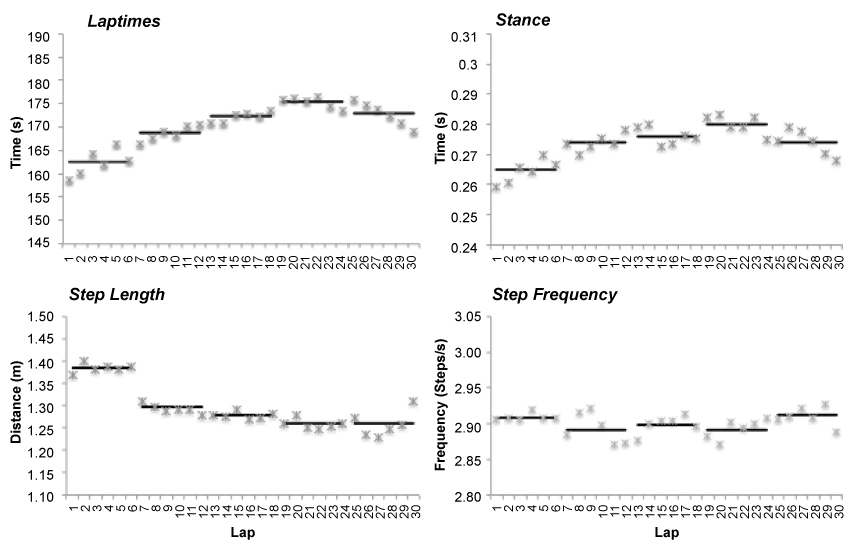
.
Figure 1: Graphs illustrate the circuit representation of corresponding laps. Circuits (lines) are the mean of the corresponding 6 laps (Star).
View Figure 1
The major gait parameters that were adjusted along with running speed were stance time and step length, while step frequency did not change throughout the run. Stance time changes followed a similar pattern of adjustment to that of running speed with a continual increase in ground contact time until the final Circuit. The importance of the increase in stance time throughout the run showed that there was also a decrease in the instantaneous running speed through the analysis area, although possibly not as significant as the average lap running speed. Connected with an increase in the stance time, runners decreased their step length throughout the run. The lack of changes in the step frequency showed that runners chose to adjust their step length to accomplish the changes in running speed. Preferred step frequency has been associated with an efficient running pattern and may indicate a runners' ability to establish this pattern even when other factors cause changes in running speed [12].
The increases in stance time and decreases in step length were similar to the changes seen in endurance race studies ranging from five km to marathon distances [1,2,6]. The lack of step frequency changes in the training run differed from the reduced stride frequency measured in racing studies incorporating both faster speeds [2] and a marathon distance [1]. This indicates that the runners in the current study may have not been challenged or fatigued to a level that elicited step frequency adjustments away from preferred.
Lower extremity joint angles at initial contact and during the stance phase have been attributed to dampening the effects of the repetitive impacts of endurance running by attenuation distribution throughout the kinetic chain. The current study identified ankle, knee and hip angles at initial contact and maximum flexion angles during stance. Knee flexion angle at initial contact and peak knee flexion during stance were the only lower extremity joints significantly adjusted throughout the 15 km training run. Runners increased knee flexion at foot contact and decreased peak knee flexion during stance creating a stiffer knee joint during the training run, which may have been adopted to maintain their preferred step frequency [13]. The increased knee stiffness may have aided in the maintenance of the running speed during the later Circuits, as it increased the stored and released energy during stance Figure 2 and Figure 3.
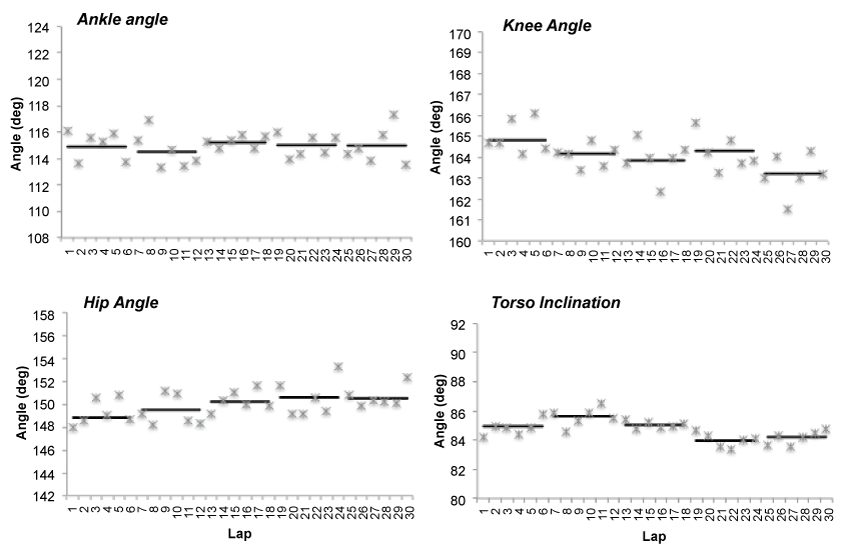
.
Figure 2: Graphs illustrate the circuit representation of corresponding laps for DVs at foot contact. Circuits (line) are the mean of the corresponding 6 laps (star).
View Figure 2
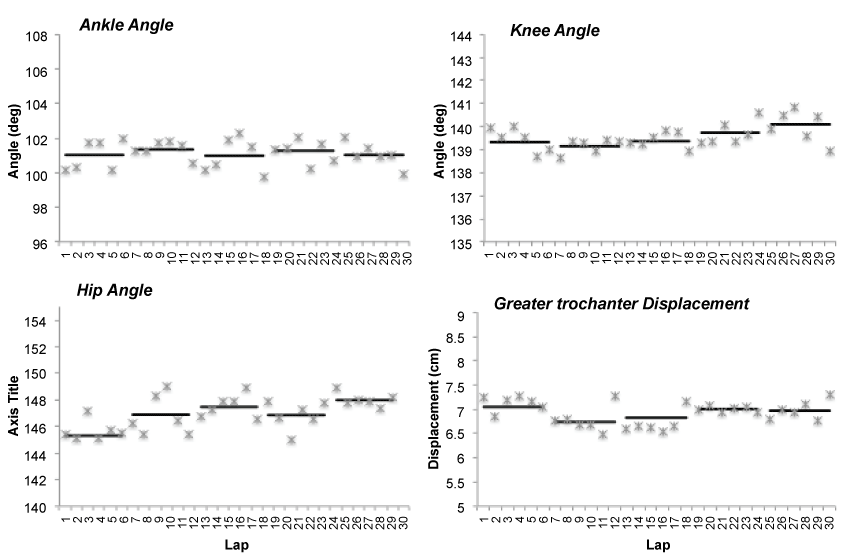
.
Figure 3: Graphs illustrate the circuit representation of corresponding laps for peak flexion during stance and greater trocharter vertical displacement. Circuits
(lines) are the mean of the corresponding 6 laps (star).
View Figure 3
The importance of the decreased torso inclination during the later circuits indicates that the runners ran with a more forward lean. Further investigation into the importance of this lean is needed, but it is theorized that it may be a mechanism to shift the systems' center of mass forward. A forward positioned center of mass limits opposition of forward momentum, which may reduce energy expenditure and oxygen consumption to maintain running speed. Additional investigation should be conducted to investigate the affects of a more forward trunk lean on breathing efficiency during endurance running. Another possible explanation of the increased torso inclination is the contraction of the hip flexors which may assist in the ability of the lower extremity to store and release energy during the stance phase.
A limitation of the two-dimensional analysis technique and kinematic marker set used for the 15 km training run is the use of greater trochanter vertical displacement as a representation of the center of mass motion. As observed with the repositioning of the body throughout the run, torso lean and joint positioning, the center of mass is not a fixed location but rather is fluid. The lack of significant difference in the vertical excursion during the stance phase, as represented by the greater trochanter vertical displacement, may be associated with the usage of a fixed bony landmark opposed to the estimated center of mass. Significant differences were expected during the 15 km training run due to the effects of knee flexion angles and speed on leg compliance [14,15].
The knowledge of the runners as to the location of the data collection and videotaping area was a limitation of the current study. The measured stance phase, step frequency and step length during the digitized frames may not have been the best representation of the overall average speeds of each lap due to the "performance for the camera" aspect observed during the runs. Runners seemed to behave more variable in their speeds and gait patterns away from the camera area then when they passed through the field of view recording area. A major goal of the study was to capture the runners in a "natural training run". The course was a half-kilometer lap, which may have not been a proper representation of the environment and terrain that the runners would naturally experience. Most of the dependent variables were extracted from the data captured at 120 Hz and manually digitized. The ability to accurately capture the frames of foot contact and toe-off allow for the possible error (up to 0.0167 s) in the temporal components. Future studies incorporating the use if inertial sensor may provide a more accurate description of changes that occur in more detail for endurance runs.
Conclusion
Runners in the current study adjusted running gait parameters and knee joint angles reflective of decreases in running speed. Two-dimensional video analysis identified runners adjusted speed, running parameters (stance time and step length), torso inclination and knee flexion at initial contact and maximal flexion during stance. Further research should investigate the lack of changes in step frequency in a non-competitive versus competitive setting and the influence of fatigue on endurance running gait parameters. Training protocols may benefit from understanding whether runners adjust their kinematics in similar ways during training and races.
Ethical Statement
All participants granted their written informed consent to participate. The informed consent document (Protocol #1403-4758) was institutionally approved by the UNLV Internal Review Board.
References
-
Chan-Roper M, Hunter I, W Myrer J, L Eggett D, K Seeley M (2012) Kinematic changes during a marathon for fast and slow runners. J Sports Sci Med 11: 77-82.
-
Hanley N, Smith LC, Bissas A (2011) Kinematic variations due to changes in pace during men's and women's 5 km road running. International Journal of Sports Science & Coaching 6: 243-252.
-
Hasegawa H, Yamauchi T, Kraemer WJ (2007) Foot strike patterns of runners at the 15-km point during an elite-level half marathon. J Strength Cond Res 21: 888-893.
-
Kasmer ME, Liu XC, Roberts KG, Valadao JM (2013) Foot-strike pattern and performance in a marathon. Int J Sports Physiol Perform 8: 286-292.
-
Larson P (2014) Comparison of foot strike patterns of barefoot and minimally shod runners in a recreational road race. Journal of Sport and Health Science3: 137-142.
-
Elliot B, Ackland T (1981) Biomechanical effects of fatigue on 10,000 meter running technique. Res Q Exerc Sport 52: 160-166.
-
James SL, Bates BT, Osternig LR (1978) Injuries to runners. Am J Sports Med 6: 40-50.
-
James CR, Dufek JS, Bates BT (2006) Effects of stretch shortening cycle exercise fatigue on stress fracture injury risk during landing. Res Q Exerc Sport 77: 1-13.
-
Fredericson M, Misra AK (2007) Epidemiology and aetiology of marathon running injuries. Sports Med 37: 437-439.
-
Gallo RA, Plakke M, Silvis ML (2012) Common leg injuries of long-distance runners: anatomical and biomechanical approach. Sports Health 4: 485-495.
-
Hanley B (2015) Pacing profiles and pack running at the IAAF World Half Marathon Championships. J Sports Sci 33: 1189-1195.
-
Mercer JA, Bezodis NE, Russell M, Purdy A, Delion D (2005) Kinetic consequences of constraining running behavior. J Sports Sci Med 4: 144-152.
-
Heiderscheit BC, Chumanov ES, Michalski MP, Wille CM, Ryan MB (2011) Effects of step rate manipulation on joint mechanics during running. Med Sci Sports Exerc 43: 296-302.
-
Chan CW, Rudins A (1994) Foot biomechanics during walking and running. Mayo Clin Proc 69: 448-461.
-
Farley CT, González O (1996) Leg stiffness and stride frequency in human running. J Biomech 29: 181-186.
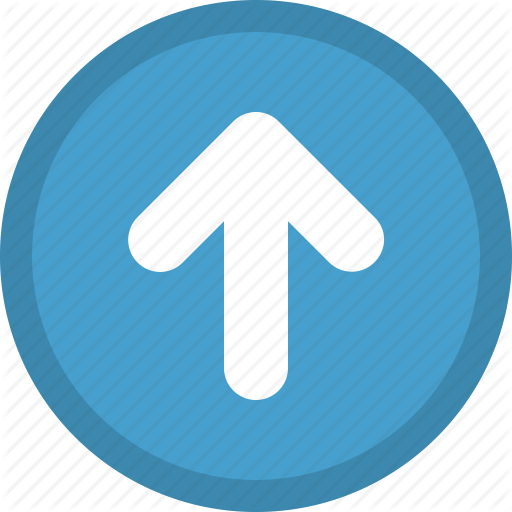