A Review: Are All Inhaled Fibres, Such as Asbestos, Toxic?
Sumayah Abdul-Jabbar* and Luigi G Martini
Institute of Pharmaceutical Science, King's College London, UK
*Corresponding author:
Sumayah Abdul-Jabbar, MPharm, Institute of Pharmaceutical Science, King's College London, 150 Stamford Street SE1 9NH, UK, Tel: 07988598580, E-mail: sumayah.abdul-jabbar@kcl.ac.uk
Int Arch Clin Pharmacol,
iacp-1-003, (Volume 1, Issue 1),
Review Article
Received: April 17, 2015: Accepted: Aug 03, 2015: Published: Aug 05, 2015
Citation: Abdul-Jabbar S, Martini LG (2015) A Review: Are All Inhaled Fibres, Such as Asbestos, Toxic?. Int Arch Clin Pharmacol 1:003
Copyright: © 2015 Abdul-Jabbar S. This is an open-access article distributed under the terms of the Creative Commons Attribution License, which permits unrestricted use, distribution, and reproduction in any medium, provided the original author and source are credited.
Abstract
Exposure to asbestos is well known to have a causal relationship with the development of lung cancer. In order to understand the mechanism of this toxicity an extensive review was undertaken. Findings were able to show that the morphology of the asbestos (which is fibrous) has a marked correlation with its toxicity. It is this morphology that mutually contributes to enhanced aerodynamic properties making asbestos ideal for deposition in the lungs as well as giving it mutagenic properties. Therefore, it is inappropriate to ignore the intrinsic property of fibres, especially due to an increase in the amount of synthetic fibres used commercially including the pharmaceutical industry. The development of fibres as medically inhaled therapies is on the increase and therefore it is very important to examine how to benefit from the enhanced aerodynamic properties whilst minimising any toxicity.
Keywords
Microfibres, Needle-shaped particles, Inhaled products, Asbestos toxicity
The use of 'needle-shaped' particles in medical and pharmaceutical industry is emerging through the use of carbon-nanotubes [1] and nanofibers [2] in particular. The use of such particles has advantages such as targeted drug delivery, enhanced bioavailability profile and fabrication of surfaces to mimic tissues for tissue engineering and regenerative medicine. However the use of nanoparticles in the form of needle-shaped fibres in particular has raised concern of toxicity due to structural resemblance to asbestos [3].
Asbestos is a group of mineral fibres of hydrated silicates with a variety of physical characteristics and metal composites (chrysotile, actinolite, amosite, anthophyllite, crocidolite, and tremolite) [4]. Asbestos fibres were frequently used in industry due to their durability, heat-resistance, and low cost. In 1987, the International Agency for Research on Cancer has designated asbestos fibres as a Group I (definite) human carcinogen [5]. This classification was coupled with the fact that inhalation of asbestos fibres due to occupational exposure resulted in an epidemic of asbestos related diseases, which included pulmonary fibrosis (asbestosis), lung cancer and malignant mesotheliomas [6].
At present the use of asbestos is banned in many countries while the use of synthetic vitreous fibres (SVF) such as fibreglass, mineral wool and ceramic fibre is tightly regulated via hazard classifications and regulations governing permissible exposure levels within workplace [7]. This increased regulation was in response to the strong correlation established between asbestos toxicity and its morphology, the needle-shaped [8].
While needle-shaped particles demonstrated promise in improving inhaled delivery for a number of drugs [9-13] one should not neglect the toxicity that their shape bears. Needle-shaped particles have profound aerodynamic properties that allow them to both deposit and persist within the lung tissue.
Needle-shaped particles are by definition elongated and hence have a high axial ratio. Such particles have an aerodynamic diameter, Dae, independent of their length [9]. It is this peculiar characteristic that enables long thin fibres to orient with the smallest diameter in the direction of air flow (align along their long axis) allowing them to deposit deep within the lungs [14]. Furthermore, elongated particles which reach alveoli can deposit there by the mechanism of interception in contrast to compact particles of the same aerodynamic diameter (≤2μm) which would be more likely to be exhaled under normal breathing conditions [10].
In order to achieve successful respiratory habitation retention need to follow the deposition and that is achieved via resisting clearance mechanism. The lung has a built in defence clearance mechanism to protect it from inhaled pathogens. However, those particles that escape the clearance mechanisms and accumulate over repeated exposure are known to be bio-persistence (biologically durable). Fibrous structures have shown to be bio-persistent [4,15].
Research using rodents exposed by inhalation to synthetic vitreous fibres have confirmed that pulmonary effects are determined by what is known as the "Three D's", dose (of fibre in the lung), dimension, and durability. Lung dose over time is determined by fibre deposition and bio-persistence in the lung. The deposition in the lung is inversely related to fibre diameter. Bio-persistence is directly related to fibre length and inversely related to fibre dissolution and fragmentation rates [7].
While asbestos is a fibre with a high axial ratio, nanofibres/nanotubes/nanowires have an even higher axial ratio due to their smaller cross sectional area and hence even better aerodynamic properties. Carbon nanotubes are of a low density and therefore produce suspended particulate matter of a respirable size. Collective results of rodent studies showed that regardless of the process by which carbon nanotubes were synthesized and the types and amounts of metals they contained, they were capable of producing inflammation, epithelioid granulomas (microscopic nodules), fibrosis, and biochemical/toxicological changes in the lungs [16].
Asbestos has been shown to escape clearance mechanism and moreover has surface properties that permit adsorption of endogenous proteins such as ferritin [17] immunoglobulin G [18] and vitronectin [19]. These complexes allow asbestos to exhibit an even greater bio-persistent [20] and hence prolonged deposition and chronic injury.
The needle-shaped particles are subjected to great physical interaction with cell molecules. Physical interactions with cells and DNA are the main principles behind the mechanism of asbestos pathology i.e. mechanical damage. [5]. It is also been shown that long, thin fibres are more active than short, thick fibres. This difference in activity may be explained as slim fibres tangle destructively with chromosomes (being of comparable size) [21]. This property is not unique to asbestos but rather it is an inherent property of any fibre [22].
Using asbestos as an example of a microfibre, it is apparent that the particle characteristic that account for enhanced lung accessibility, habitation and pathology is mutual. Consequently it is inappropriate to disregard the intrinsic toxicity of needle-shaped particles when developing inhaled therapy. Therefore by examining each phenomenon it may reveal how this morphology can be manipulated to enhance drug delivery whilst minimising toxicity.
Human diseases associated with exposure to asbestos fibres are either inflammatory (fibrosis) or malignant (cancer) in nature [4]. Carcinogenesis is a complex multi-mode disease with variety of dynamics that can lead to uncontrolled proliferation of the body's own cells [23]. It may be a direct genetic mutation [24], a mitotic spindle interaction [4] or an epigenetic influence [24]. While asbestos induced inflammation is typically chronic with prolonged inflammatory mediators' release which in turn, has a great potential to stimulate cell proliferation and possibly facilitating malignant transformation [25]. In addition, reactive oxygen species may also play an important role in the mutagenic pathology. Therefore It is not a simple "one-hit" mechanism and more interestingly scientific studies revealed that asbestos can induce carcinogenicity by all these mechanism [4,23,26].
The simplest mechanism is via direct genetic mutation (Figure 1). It involves asbestos fibres penetrating the target cell and interacting physically with DNA leading to mutations. Depending upon what genes these events affect, the cell carrying the aberrations can die or survive as a normal or abnormal cell (tumour). Tumours form when critical genes, oncogenes and regulatory genes (tumour suppressor genes), are mutated. They lead to the cell to lose the capability to differentiate, to control its own growth, and to function normally constituting the initiation step in the carcinogenic process [26]. There is overwhelming evidence to support the concept that most cancers develop as the consequence of mutations in critical genes [27].
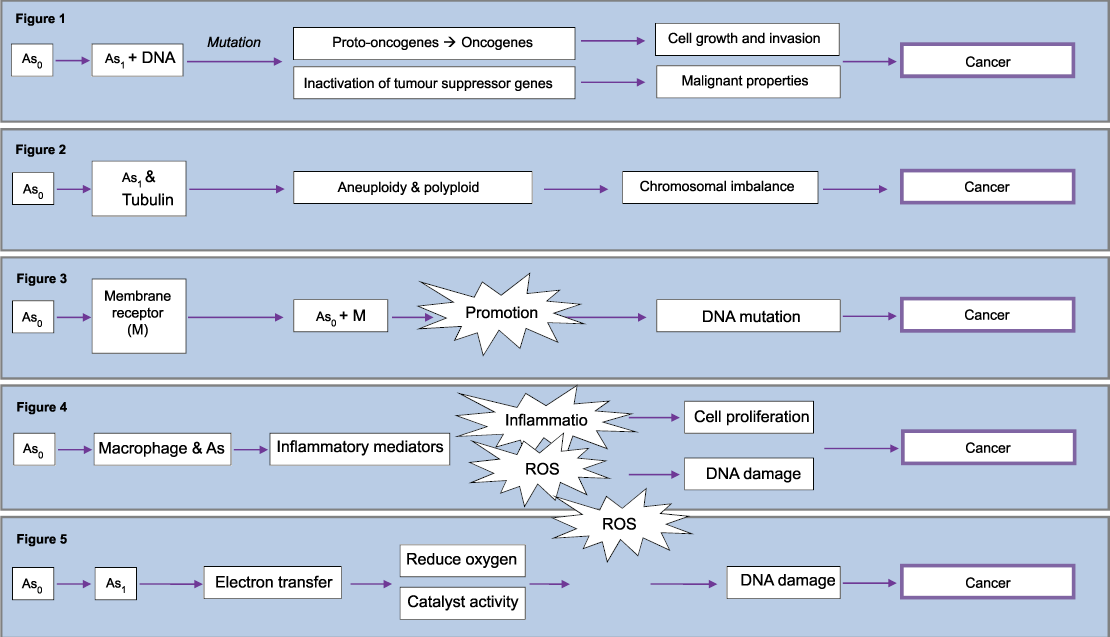
.
Figure 1: Mechanism for direct genetic mutation of asbestos
Figure 2: Mechanism of asbestos mitotic spindle interaction
Figure 3: Epigenetic mechanism of asbestos
Figure 4: Mechanism of asbestos induced Inflammation
Figure 5: Reactive oxygen species formation by asbestos
View Figure 1
Human mesotheliomas have been associated with a number of direct genetic mutations. This includes monosomy of chromosomes 3 and 13 which both have a tumour suppressor gene [28-30]. In addition, extra copies of chromosome 7 were also found, it is the site of the proto-oncogene HER-1 that encodes the epidermal growth factor receptor (EGF-R) [31,32]. Extra copies mean that there is amplification and over expression of proto-oncogenes leading to cell proliferation [26].
Asbestos fibres are long, rigid and bio-persistent making them capable of binding to tubulin, a protein that makes up the spindle apparatus necessary for chromosomal separation during cell division, inducing aneuploidy and polyploidy (Figure 2) [26]. This is a result of physical interactions of the fibres with the mitotic spindle, leading to chromosomal breakage, loss, or improper chromosomal segregation during mitosis [4].
A number of studies have managed to explain these numerical chromosomal abnormalities. In aneuploidy, cells exposed to fibres showed to have lagging chromosomes, bridges, and sticky chromosomes in anaphase [33]. While in vitro, asbestos fibres have been shown to adhere to chromosomes in metaphase and cause polyploidy [34]. Human mesothelial cells had high incidence of chromosomal abnormalities confirming that this mechanism accounts for carcinogenicity [35].
Cancer is both an epigenetic and a genetic disease. Epigenetic alterations in cancer often serve as potent surrogates for genetic mutations. Epigenetic is defined as modifications of DNA or associated factors that have information content [36]. Gene silencing (an epigenetic modification) has been witnessed with asbestos fibre and is considered as an indirect mechanism for carcinogenicity [37].
Figure 3 illustrates a simplified mechanism of epigenetic which is a promotional activity of asbestos. Asbestos binds to membrane sites and causes epigenetic effects that initially alter cellular growth and function and later lead to carcinogenicity as a consequence of cell proliferation [23,26].
Macrophages attempt to phagocytise fibres that are not removed by mucociliary clearance. As macrophages range in diameter from 10 to 20μm, shorter fibres are more likely to be completely phagocytised by alveolar macrophages than longer fibres. Those longer ones lead to incomplete or 'frustrated' phagocytises, which is characterized by prolonged production of cytokines and reactive oxygen species (ROS) [15]. This inflammatory environment produces injury and proliferation of lung epithelial cells, (Figure 4) [38].
ROS are superoxide free radicals and hydrogen peroxide. ROS can damage cellular macromolecules via lipid peroxidation, oxidative DNA damage [39] and by acting as a secondary messenger to alter signal transduction pathways and gene expression [35]. DNA damage manifest as oxidised bases, DNA single strand break, DNA double strands breaks, mutations and numerical and structural chromosomal aberrations [40,41].
In addition to the indirect formation of ROS during inflammation it can also be formed directly by asbestos (Figure 5). There is the potential of transfer of an electron from the asbestos fibre to cellular molecules leading to the formation of a ROS. This initiation of redox cycling is an attribute of the redox-active transition metals of asbestos [42]. A study showed that ferrous iron in certain asbestos fibres reduced oxygen to form a super oxide free radical [43]. A second study showed asbestos fibres can catalyse the formation of both the superoxide radical and hydroxyl radical from hydrogen peroxide [44]. Some studies proved those mechanisms via the use of scavengers of active oxygen species, such as polyethylene glycol (PEG)-conjugated catalase [45] and dismutase [46]. Those scavengers were able to prevent inflammation both in vivo and in vitro [47].
A very recent publication reveals that in addition to the physical mechanism of asbestos it also has a very interesting optical mechanism that can interfere with mitosis. Fibres with specific dimensions can transmit both UVA and UVB [48]. Long mitochondria on microtubules may be able to act as UV-lasers suggesting some universal secret role for UV related to mitosis [49]. Therefore, the insertion of fibre "short-circuits" could then cause upsets in mitosis control, and hence DNA irregularities [48].
Relevance to Pharmaceutics
While there are a number of inhaled drugs that have been delivered as needle-shaped particles there is also those that are intended for other routes of administration. It is equally important to consider the toxicity in such instances especially if they are liable to inhalation during handling.
Drugs that exhibit high solubility will absorb water preferentially upon entry into the respiratory tract. Therefore these highly soluble drugs will not conserve their needle-shape and instead will ultimately retrieve into spherical droplets instead [50].
Sodium nedocromil is used for prophylaxis of asthma. It is available commercially as a trihydrate which is highly hygroscopic. When the particles are inhaled, they take up moisture in the humid environment of the respiratory tract and thus increase their particle size (by hygroscopic growth) which adversely affects deposition in the lung. Nedocromil is prepared typically by crystallisation yielding elongated respirable particles which have a very low solubility and hence no longer subject to hygroscopic growth. Therefore nedocromil had an improved lung deposition in comparison to its sodium salt [9].
An identical scenario was illustrated by cromolyn sodium which is also indicated for prophylaxis of asthma. Cromolyn sodium is highly hygroscopic while the parent acid, cromoglycic acid, has a very low solubility in water and an elongated shape making it a much better candidate for respiratory deposition [10].
Toxicity profile for sodium nedocromil and cromolyn sodium has been published which both illustrated a minimal systematic toxicity [51,52]. However, it is unknown for nedocromil and cromoglycic acid. In addition it is also important to use crystallisation solvents that are inert so that their residuals do not induce acute toxicity [9].
Another potential advantage of elongated particles is their larger drug mass carrying [9]. This means for the same aerodynamic diameter, a fibre like particle carries a larger drug mass than a compact one. Therefore, the total number of particles to be delivered for the same effect in therapy would be lower with elongated shapes. This could be advantageous because of greater colloidal stability of the preparation with the fibre-like particles, due to lower interfacial energy [10]. As a result, needle-shaped serves as ideal carries for dry powder inhalers (DPI).
The most common formulation approach of DPI is to blend the drug with a larger inert carrier (e.g. lactose). This will overcome the cohesive forces between the micronized drug particles and improve flow. During inhalation manoeuvre the drug should be liberated from the carrier in order to penetrate into the lungs [53]. These performances in turn are highly dependent on particle-particle interaction or more precisely drug-carrier interactions [13]. This lead to a significant research in DPI carrier technology with a special focus on the influence of morphology [54,55].
In one study α-Lactose monohydrate were prepared to have different morphological features but with similar particle size. Each batch of lactose was mixed in the same manner with micronized salbutamol sulphate. The fine particle fraction and dispersibility of salbutamol sulphate significantly increased with elongation ratio (length/width) of lactose crystals after aerosolisation of the formulations [13].
While in another study, both drug (salbutamol sulphate) and carrier (lactose) morphology was examined. Increasing the elongation ratio of the carrier or drug improved the deposition profiles of salbutamol sulphate, suggesting that the more elongated particles would be more aerodynamic and favour deep lung penetration. However, interestingly, as the elongation ratio increased the flow properties of the carrier were affected detrimentally. This flaws the purpose of the carrier and hence reduced the content uniformity of salbutamol sulphate and drug emission from the inhaler device [11].
Furthermore, a study was conducted aiming at improving dry powder inhalation behaviour of steroid KSR-592 with lactose by altering the crystal shape of the drug rather than the carrier. Platelet like (form α) was found to have strong cohesive and adhesive properties making aerosolization of the drug difficult. Needle-shaped crystals of the steroid (form β) were obtained by the polymorphic transformation with the aim to overcome these undesirable properties [12]. The hypothesis behind that was that the contact state between fibrous particles is extremely unstable promoting excellent de-aggregation properties [56]. This hypothesis has proved right as the respirable fraction of the β form was significantly higher, about 6 folds more, than that of the α form using a Diskhaler® [12]. In a separate paper, this result was criticised as the relative change in performance may be difficult to be directly attribute to morphology as the steroid underwent polymorphic transformation [53].
Recommendations for Developing Needle-Shaped Inhaled Therapies
After considering both sides of the case, the toxicity mechanism and the enhanced aerodynamic properties of the needle-shaped particles one can discuss the potential of developing new inhaled therapies consisting of fibres. Can fibres enhanced aerodynamic properties be exploited in optimised lung drug delivery?
Asbestos itself has demonstrated a desired clinical application in nucleic acid delivery (gene therapy). This was achieved by asbestos behaving as a transfecting agent to insert nucleic acid into the genome. Studies showed that asbestos managed to insert plasmid DNA vector into cos 7 monkey cells [57] and viral DNA into both liver cells and mouse fibroblast cell [58].
Asbestos is a natural fibre with well-defined properties. Therefore unlike fibres for therapy which are prepared synthetically and hence more amenable to modification before use [4]. This fundamental difference can permit the safe use of fibres. Asbestos itself showed a decreased in binding capability to proteins e.g. bovine serum albumin when heated. In addition heat treated asbestos had reduced cellular cytotoxicity than untreated. One possible mechanism behind this reduce toxicity is the escape of electrons within the mineral upon heating [59]. Consequently this will prevent the formation of ROS.
Surface properties of a synthetic fibre can be selected and designed in a number of approaches to reduce toxicity. Eliminating the use of materials that have redox-active metals [4], modifying surface oxidising properties [59], charge and chemical modification to determine the location and degree of adsorption [60] and treatment of fibres to make them more soluble (improving their dispersion in biological fluids) and therefore reducing bio-persistent [60] are few of the approaches possible.
Biologically durability also accounted for asbestos pathology. Experimental data clearly indicate that fibres that are difficult or impossible to eliminate by the pulmonary clearance mechanisms, and that are not subject to physical breakdown or chemical dissolution, are more likely to exert their bioactive effects and are, thus, potentially more toxic [60]. Consequently manufacturing of fibres that are more subjected to clearance will reduce toxicity dramatically.
The rate at which fibres are physically cleared from the alveolar gas exchange region of the lung depends upon the ability of macrophages to engulf them. Fibres that are longer than the macrophages will not be engulfed. Asbestos breakage occurs longitudinally, resulting in additional fibres of the same length but smaller diameter. However this is not the case with amorphous fibres as they break perpendicular to their long axis resulting in fibres that can be engulfed by the macrophages [59]. Therefore they are less bio-persistent than asbestos and hence less toxic.
A study looked at the effects of fibre dimension and solubility on bio-persistence. In the study intratracheal injection of nine different fibres were studied. The relative bio-persistence of fibres was assessed from the changes in mean lung burden, as determined by electron microscopy post injection. In line, the ability of the test materials to resist dissolution was measured using a simple in vitro experiment. The observed differences in the persistence of fibres of differing length recovered from rat lungs were consistent with the current hypothesis that short fibres are cleared by cellular processes and long fibres by dissolution and disintegration. Differences in persistence of long (>20mm) fibres were correlated with measured rates of dissolution in vitro [61].
The European Union (EU) has proposed a series of protocols for the in vivo examination of man-made vitreous fibres. The European Commission Joint Research Centre published standardised methods regarding the evaluation of both acute and chronic exposures to man-made fibres. Those standardised methods give information regarding test animals, exposure levels (dose) along with duration and frequency, observational period, data collection and statistical analysis. Also criteria were established for the classification and labelling of synthetic mineral fibres.
This European Commission Directive was derived based upon an extensive program evaluating current scientific knowledge on fibre pathogenicity and its relationship to the bio-persistence of long fibres. Within this context, the bio-persistence of fibres longer than 20μm was found to be a good predictor of the lung burden and early pathological changes in chronic inhalation studies with fibres as well as of the tumour response in chronic intraperitoneal studies. The analysis that provided the scientific basis for the relationship of bio-persistence to the chronic inhalation results is presented in detail. Proportional odds regression techniques were used to determine the relationship between both inhalation and intratracheal instillation bio-persistence clearance half-times and the collagen deposition at the broncho-alveolar junction. The results indicate all the indicators of bio-persistence considered are equally good predictors of the early long-term change that occurs in the lung in response to more durable fibres. This change the collagen deposition at the broncho-alveolar junction is a precursor of interstitial fibrosis, which has been shown to be associated with tumour response in fiber-exposed animals [62,63].
The known toxicity mechanism of asbestos is on a molecular and cellular level i.e. biological mechanism. For developing a pharmacotherapy then it would be essential to understand the pharmacokinetics and pharmacodynamics of the molecule, from both a physical and a chemical aspect. This should be drug specific in order to assess elimination and target cell specificity [26].
The surface, clearance and breakage modifications of synthetic microfibers coupled to the opportunity to evaluate activity prior to wide scale use is a foundation for future applications. Therefore the aptitude of fibres in enhanced lung delivery should not be neglected. Instead should recognise that their use must be selective and balanced against their potentially harmful inherent properties.
In conclusion, the current understanding of asbestos pathology is a cooperative framework to guide the design and post-processing of inhaled microfibers. This framework should be employed in a pre-emptive attempt to reduce the potential for exposure-related 'fibre-diseases' by undertaking the necessary safety and toxicology studies prior to wider use.
References
-
He H, Pham-Huy LA, Dramou P, Xiao D, Zuo P, et al. (2013) Carbon nanotubes: applications in pharmacy and medicine. Biomed Res Int 2013: 578290.
-
Liu W, Thomopoulos S, Xia Y (2012) Electrospun nanofibers for regenerative medicine. Adv Healthc Mater 1: 10-25.
-
Lam CW, James JT, McCluskey R, Arepalli S, Hunter RL (2006) A review of carbon nanotube toxicity and assessment of potential occupational and environmental health risks. Crit Rev Toxicol 36: 189-217.
-
Sanchez VC, Pietruska JR, Miselis NR, Hurt RH, Kane AB (2009) Biopersistence and potential adverse health impacts of fibrous nanomaterials: what have we learned from asbestos? Wiley Interdiscip Rev Nanomed Nanobiotechnol 1: 511-529.
-
Toyokuni S (2009) Mechanisms of asbestos-induced carcinogenesis. Nagoya J Med Sci 71: 1-10.
-
Meeks ME (1982) Pathogenesis of asbestos-associated diseases. N Engl J Med 307: 1526-1527.
-
Hesterberg TW, Hart GA (2001) Synthetic vitreous fibers: a review of toxicology research and its impact on hazard classification. Crit Rev Toxicol 31: 1-53.
-
Bertrand R, Pezerat H (1980) Fibrous glass: carcinogenicity and dimensional characteristics. IARC Sci Publ : 901-911.
-
Chan HK, Gonda I (1995) Physicochemical characterization of a new respirable form of nedocromil. J Pharm Sci 84: 692-696.
-
Chan HK, Gonda I (1989) Respirable form of crystals of cromoglycic acid. J Pharm Sci 78: 176-180.
-
Larhrib H, Martin GP, Marriott C, Prime D (2003) The influence of carrier and drug morphology on drug delivery from dry powder formulations. Int J Pharm 257: 283-296.
-
Ikegami K, Kawashima Y, Takeuchi H, Yamamoto H, Isshiki N, et al. (2002) Improved inhalation behavior of steroid KSR-592 in vitro with Jethaler by polymorphic transformation to needle-like crystals (beta-form). Pharm Res 19: 1439-1445.
-
Zeng XM, Martin GP, Marriott C, Pritchard J (2000) The influence of carrier morphology on drug delivery by dry powder inhalers. Int J Pharm 200: 93-106.
-
Timbrell V (1982) Deposition and retention of fibres in the human lung. Ann Occup Hyg 26: 347-369.
-
Moolgavkar SH1, Brown RC, Turim J (2001) Biopersistence, fiber length, and cancer risk assessment for inhaled fibers. Inhal Toxicol 13: 755-772.
-
Lam CW, James JT, McCluskey R, Arepalli S, Hunter RL (2006) A review of carbon nanotube toxicity and assessment of potential occupational and environmental health risks. Crit Rev Toxicol 36: 189-217.
-
Fubini B, Barceló F, Otero Areán C (1997) Ferritin adsorption on amosite fibers: possible implications in the formation and toxicity of asbestos bodies. J Toxicol Environ Health 52: 343-352.
-
Donaldson K, Hill IM, Beswick PH (1995) Superoxide anion release by alveolar macrophages exposed to respirable industrial fibers - modifying effect of fiber opsonization. Exp Toxicol Pathol 47: 229-231.
-
Wu J, Liu W, Koenig K, Idell S, Broaddus VC (2000) Vitronectin adsorption to chrysotile asbestos increases fiber phagocytosis and toxicity for mesothelial cells. Am J Physiol Lung Cell Mol Physiol 279: 916-923.
-
Ghio AJ, Stonehuerner J, Richards J, Devlin RB (2008) Iron homeostasis in the lung following asbestos exposure. Antioxid Redox Signal 10: 371-377.
-
Oshimura M, Hesterberg TW, Tsutsui T, Barrett JC (1984) Correlation of asbestos-induced cytogenetic effects with cell transformation of Syrian hamster embryo cells in culture. Cancer Res 44: 5017-5022.
-
De Vuyst P, Dumortier P, Swaen GM, Pairon JC, Brochard P (1995) Respiratory health effects of man-made vitreous (mineral) fibres. Eur Respir J 8: 2149-2173.
-
Barrett JC (1994) Cellular and molecular mechanisms of asbestos carcinogenicity: implications for biopersistence. Environ Health Perspect 102 Suppl 5: 19-23.
-
Mossman B, Light W, Wei E (1983) Asbestos: mechanisms of toxicity and carcinogenicity in the respiratory tract. Annu Rev Pharmacol Toxicol 23: 595-615.
-
Manning CB, Vallyathan V, Mossman BT (2002) Diseases caused by asbestos: mechanisms of injury and disease development. Int Immunopharmacol 2: 191-200.
-
Voytek P, Anver M, Thorslund T, Conley J, Anderson E (1990) Mechanisms of asbestos carcinogenicity. J Am Coll Toxicol 9: 541-550.
-
Barrett JC (1993) Mechanisms of multistep carcinogenesis and carcinogen risk assessment. Environ Health Perspect 100: 9-20.
-
Zbar B (1989) Chromosomal deletions in lung cancer and renal cancer. Important Adv Oncol .
-
Flejter WL, Li FP, Antman KH, Testa JR (1989) Recurring loss involving chromosome-1, chromosome-3, and chromosome-22 in malignant mesothelioma: possible sites of tumor suppressor genes. Genes Chromosomes Cancer 1: 148-154.
-
Bishop JM (1991) Molecular themes in oncogenesis. Cell 64: 235-248.
-
Olofsson K, Mark J (1989) Specificity of asbestos-induced chromosomal aberrations in short-term cultured human mesothelial cells. Cancer Genet Cytogenet 41: 33-39.
-
Carpenter G (1984) Properties of the receptor for epidermal growth factor. Cell 37: 357-358.
-
Hesterberg TW, Barrett JC (1985) Induction by asbestos fibers of anaphase abnormalities: mechanism for aneuploidy induction and possibly carcinogenesis. Carcinogenesis 6: 473-475.
-
Wang NS, Jaurand MC, Magne L, Kheuang L, Pinchon MC, et al. (1987) The interactions between asbestos fibers and metaphase chromosomes of rat pleural mesothelial cells in culture. A scanning and transmission electron microscopic study. Am J Pathol 126: 343-349.
-
Gibas Z, Li FP, Antman KH, Bernal S, Stahel R, et al. (1986) Chromosome changes in malignant mesothelioma. Cancer Genet Cytogenet 20: 191-201.
-
36. Iacobuzio-Donahue CA1 (2009) Epigenetic changes in cancer. Annu Rev Pathol 4: 229-249.
-
Esteller M (2005) Dormant hypermethylated tumour suppressor genes: questions and answers. J Pathol 205: 172-180.
-
Shukla A, Gulumian M, Hei TK, Kamp D, Rahman Q, et al. (2003) Multiple roles of oxidants in the pathogenesis of asbestos-induced diseases. Free Radic Biol Med 34: 1117-1129.
-
Kamp DW, Weitzman SA (1999) The molecular basis of asbestos induced lung injury. Thorax 54: 638-652.
-
Jaurand MC (1996) Use of in-vitro genotoxicity and cell transformation assays to evaluate the potential carcinogenicity of fibres. IARC Sci Publ : 55-72.
-
Okayasu R, Takahashi S, Yamada S, Hei TK, Ullrich RL (1999) Asbestos and DNA double strand breaks. Cancer Res 59: 298-300.
-
Bernstein D, Castranova V, Donaldson K, Fubini B, Hadley J, et al. (2005) Testing of fibrous particles: short-term assays and strategies. Inhal Toxicol 17: 497-537.
-
Wong SF, Halliwell B, Richmond R, Skowroneck WR (1981) The role of superoxide and hydroxyl radicals in the degradation of hyaluronic acid induced by metal ions and by ascorbic acid. J Inorg Biochem 14: 127-134.
-
Weitzman SA, Graceffa P (1984) Asbestos catalyzes hydroxyl and superoxide radical generation from hydrogen peroxide. Arch Biochem Biophys 228: 373-376.
-
Mossman BT, Marsh JP, Hardwick D, Gilbert R, Hill S, et al. (1986) Approaches to prevention of asbestos-induced lung disease using polyethylene glycol (PEG)-conjugated catalase. J Free Radic Biol Med 2: 335-338.
-
Mossman BT, Marsh JP, Shatos MA (1986) Alteration of superoxide dismutase activity in tracheal epithelial cells by asbestos and inhibition of cytotoxicity by antioxidants. Lab Invest 54: 204-212.
-
Mossman BT, Marsh JP, Shatos MA (1986) Alteration of superoxide dismutase activity in tracheal epithelial cells by asbestos and inhibition of cytotoxicity by antioxidants. Lab Invest 54: 204-212.
-
Traill RR (2011) Asbestos as 'toxic short-circuit' optic-fibre for UV within the cell-net: - Likely roles and hazards for secret UV and IR metabolism. J Phys Conf Ser 329: 012017.
-
Thar R, Kühl M (2004) Propagation of electromagnetic radiation in mitochondria? J Theor Biol 230: 261-270.
-
Hickey AJ (1992) Drugs and the pharmaceutical sciences vol. 54. Pharmaceutical inhalation aerosol technology. Marcel Dekker, Inc.: New York, USA, Basel, Switzerland. Illus 365.
-
King HC (1992) Mast cell stabilizers. Otolaryngol Head Neck Surg 107: 841-844.
-
Clark B, Clarke AJ, Bamford DG, Greenwood B (1986) Nedocromil sodium preclinical safety evaluation studies: a preliminary report. Eur J Respir Dis Suppl 147: 248-251.
-
Adi H, Traini D, Chan HK, Young PM (2008) The influence of drug morphology on aerosolisation efficiency of dry powder inhaler formulations. J Pharm Sci 97: 2780-2788.
-
Young PM, Edge S, Traini D, Jones MD, Price R, et al. (2005) The influence of dose on the performance of dry powder inhalation systems. Int J Pharm 296: 26-33.
-
Zeng XM, Martin GP, Marriott C, Pritchard J (2000) The effects of carrier size and morphology on the dispersion of salbutamol sulphate after aerosolization at different flow rates. J Pharm Pharmacol 52: 1211-1221.
-
Otsuka A, Iida K, Danjo K, Sunada H (1988) Measurement of the adhesive force between particles of powdered materials and a glass substrate by means of the impact separation method .3. Effect of particle-shape and surface asperity. Chem Pharm Bull 36: 741-749.
-
Appel JD, Fasy TM, Kohtz DS, Kohtz JD, Johnson EM (1988) Asbestos fibers mediate transformation of monkey cells by exogenous plasmid DNA. Proc Natl Acad Sci U S A 85: 7670-7674.
-
Dubes GR, Mack LR (1988) Asbestos-mediated transfection of mammalian cell cultures. In Vitro Cell Dev Biol 24: 175-182.
-
Searl A (1994) A review of the durability of inhaled fibers and options for the design of safer fibers. Ann Occup Hyg 38: 839-855.
-
Fubini B (1997) Surface reactivity in the pathogenic response to particulates. Environ Health Perspect 105 Suppl 5: 1013-1020.
-
Searl A, Buchanan D, Cullen RT, Jones AD, Miller BG, et al. (1999) Biopersistence and durability of nine mineral fibre types in rat lungs over 12 months. Ann Occup Hyg 43: 143-153.
-
Moolgavkar SH, Turim J, Brown RC (2001) The power of the European Union protocol to test for carcinogenicity of inhaled fibers. Regul Toxicol Pharmacol 33: 350-355.
-
Bernstein DM, Sintes JMR, Ersboell BK, Kunert J (2001) Biopersistence of synthetic mineral fibers as a predictor of chronic inhalation toxicity in rats. Inhal Toxicol 13: 823-849.
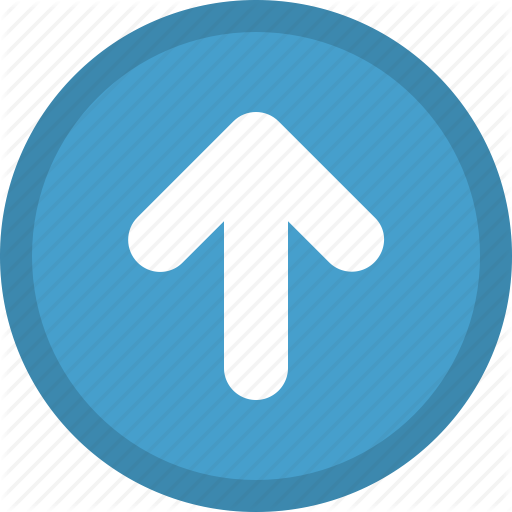