International Journal of Ophthalmology and Clinical Research
Next Generation Artificial Eyes with Dynamic Iris
Jerome Lapointe1,2*, Jean-Sébastien Boisvert1,2 and Raman Kashyap1,2,3
1Department of Engineering Physics, FABULAS Laboratory, Polytechnique Montreal, Canada
2Poly-Grames Research Center, 2500, Chemin Polytechnique, Montreal, H3T 1J4, Canada
3Department of Electrical Engineering, Polytechnique Montreal, Canada
*Corresponding author: Jerome Lapointe, FABULAS Laboratory, Polytechnique Montreal C.P. 6079, Succ. Centre-ville, Montreal (Quebec), H3C 3A7, Canada, Tel: +1 514 340 4711 (Ext 7084), Fax: +1 514 340 3218, E-mail: jerome.lapointe@polymtl.ca
Int J Ophthalmol Clin Res, IJOCR-3-062, (Volume 3, Issue 4), Review Article; ISSN: 2378-346X
Received: May 10, 2016 | Accepted: August 26, 2016 | Published: October 01, 2016
Citation: Lapointe J, Jean-Sébastien B, Kashyap R (2016) Next Generation Artificial Eyes with Dynamic Iris. Int J Ophthalmol Clin Res 3:062. 10.23937/2378-346X/1410062
Copyright: © 2016 Lapointe J, et al. This is an open-access article distributed under the terms of the Creative Commons Attribution License, which permits unrestricted use, distribution, and reproduction in any medium, provided the original author and source are credited.
Abstract
The eye is a vital organ for vision and an important element of facial expression, but also as the first point of contact between people. The loss of an eye has a far-reaching impact on an individual's psyche. Additionally, it affects a patient's professional and social life. Cosmetic rehabilitation with custom made ocular prostheses gives such individuals social and professional acceptance and mitigates problems of re-integration in society. For centuries, ocularists and eye surgeons have worked together to try to create an artificial eye as close to a realistic one as possible. All efforts and investments to improve the appearance of ocular prostheses are neutralized by the immobility of the pupil.
Several researchers have proposed ideas to solve this problem, for example, with the use of liquid crystals, sources of light, LED displays, photochromics, magnetic and polarized materials. However, none of these inventions has shown real success so far. This article summarizes the constraints arising from the ocular prostheses manufacturing process and briefly reviews past works on creating a dynamic pupil in order to understand their failures. It also describes in detail recent works and new results from our group that seem to provide a functional solution with emphasis on the challenges and opportunities for research.
Keywords
Ocular prosthesis, Prosthetic eye, Artificial eye, Iris, Pupil, LC, LCD
Introduction
The eye is a vital organ not only enabling sight but also because it is indispensable element of facial expression. The loss of an eye has an important impact on an individual's self-esteem, equally affecting social and professional interactions [1]. A very realistic artificial eye, with a hand-painted iris inside a molded ocular prosthesis and its implant sewn directly into the ocular tissues in order to follow the same movements as the healthy eye, has been the solution for most patients who have received a single prosthetic implant to replace an eye, in order to preserve their psychological well-being. However, the realistic appearance of these prostheses is limited by the pupils' immobility.
Figure 1 is a photograph of a man who possesses an ocular prosthesis instead of his left eye. Even if ambient lighting is not extreme, the difference between the two pupils is distinguishable enough to distract a speaker-listener and possibly lead to an embarrassing situation. The ultimate objective facing scientists, engineers, ocularists and eye surgeons is to create an ocular prosthesis designed with a pupil of varying dimension, in accordance to the ambient lighting.
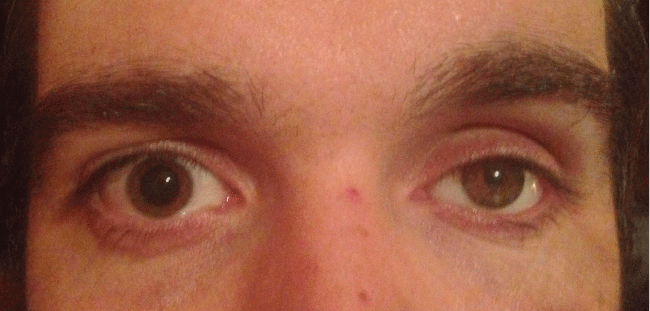
.
Figure 1: A patient possesses an ocular prosthesis in his left eye. The dissemblance between the two pupils is easily discernable. (Photo courtesy JL).
View Figure 1
The following article will first present a history of ocular prostheses and their evolution. Afterwards, in order to properly understand the constraints surrounding the design of ocular prostheses, the steps involved in their fabrication will be described. Additionally, this article will also present the existing work completed surrounding dynamic pupils, as well as the reasons behind why these devices were never commercialised. Finally, a recent technology, which would likely be a functional solution, is presented in more detail.
A History of Ocular Prostheses
The evolution of ocular prostheses
In accordance with popular belief, the first artificial eyes were made by the Egyptians (2613-2494 B.C.E). Civilisations such as the Babylonians and the Samarians most likely have also used the art of artificial eyes in their statues and mummification practices, using precious stones, silver or met al [2-5].
In 1561, Paré of Paris published a book describing two types of ocular prostheses; one that was placed under the eyelid, named "hyplepharon", and the other situated on the outside, ''celepharon'' [6]. He equally used glass and porcelain in order to construct his prostheses, which was a significant step in this science. He hence introduced the prosthesis as a shell instead of a sphere [7].
At the beginning of the 19th century, France became the center for the fabrication of ocular prostheses. It was only in 1849, thanks to Boissonneau, that the term ocularist came to be [8]. Boissonneau was producing glass eyes that were quite popular in Europe and America.
In 1853, Ludwig Muller Uri used a new material and a new method to make glass eyes for human patients. These doll eyes had a human appearance. He developed a unique method that could colour the iris. His nephew, Friedrich A. Muller is credited for developing the double glass prosthesis [9]. Peter Gouglemann, Boisonneau's student, founded a studio dedicated to ocular prostheses in 1851, in New York. During the Second World War, the glass shortage brought on the use of acrylic, used most typically in dentistry, and methyl methacrylate (MMA). The first international ocularist organisation was dubbed "American Society of Ocularists" which took shape on the 13th of October 1958. Many breakthroughs and improvements have been made in the last five decades, both in terms of material and technique [9].
Modern ocular prostheses
Most ocular prostheses made today are in the shape of a half-shell and are placed on top of an ocular muscle implant. The first to integrate an implant was Mules, in 1884 [10]. The first materials used in order to make these implants were glass, plastic, cartilage and silicone [11-13].
Towards the end of the 1940s and early 1950s, many types of implants were developed. Despite the demonstration of excellent mobility in these implants, the majority would lead to necrosis, an infection or exposure, which ultimately would lead to their removal [14].
Perry was the first to introduce implants made of hydroxyapatite. This material is biocompatible, non-toxic and contains pores that are of 500 μm in diameter. This structure would allow tissues to grow within the implant, which would substantially diminish the possibility of migration [15,16]. Polyethylene implants with high porosity density were introduced by Karesh and Dresner [17]. Despite the ocular tissues proving a slower growth rate in the porous polyethylene than in the hydroxyapatite, the polyethylene has the advantage of being less expensive and easier to manipulate [18,19]. These days, spherical and porous implants are gaining popularity.
The patient and eye surgeon satisfaction rates in response to ocular prostheses are presented in figure 2. Eye surgeons are generally less satisfied than their patients. Regardless, the global satisfaction rate on the 78 patients studied is of 71.8% [20].
Ocular Prostheses Fabrication
This subsection presents the important points surrounding the theory of ocular prostheses which would be useful in the conception of a device that could allow for a pupil with a light sensitive diameter. We will begin by presenting the various implant models that lead the trend in the shapes of ocular prostheses. Each step in the fabrication of these prostheses is equally explained. The important step in this process is the baking at a temperature of 120°C under a pressure of 300 kPa for a period of an hour. This step leads to many constraints on the conception of an ocular device.
Ocular implants
A popular model of ocular implant is a ball made of acrylic, a material that is biologically compatible, more or less spherical depending on the model, see figure 3. As indicated in figure 4, these implants have a smooth surface with interconnected tunnels of 1 to 2 mm in diameter, allowing the direct soldering of the muscles and the tissue growth. Figure 5 demonstrates, between the four implant models, the way that the tissues will invade the implant. Migration, the forward displacement and stretching of tissue, is then predicted. Given that they are permanently smooth, the implants will never compromise the covering tissue. The shape of the implant through the tissues is visible. With the help of implants to the right of the figures, the external prosthesis possesses better movement. Indeed, with the irregular surface of the implants, the prosthesis can fit more easily, which helps it remain in place when the muscles activate the implant.
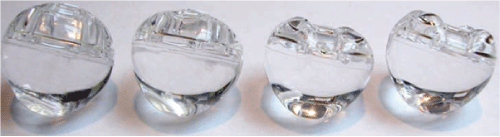
.
Figure 3: Four types of ocular implants (Photo courtesy Jean-François Durette, ocularist).
View Figure 3
The porous implant, shown in figure 6, becomes increasingly more common. It allows for fibrovascular growth everywhere inside of the implant, which is what ultimately allows better muscle movement [22]. Given its spherical shape, this implant uses the ocular prosthesis depicted on the left, as shown on figure 7.

.
Figure 5: Tissue growth after the direct soldering of muscles on the four implant models. Adapted from Durette [21].
View Figure 5
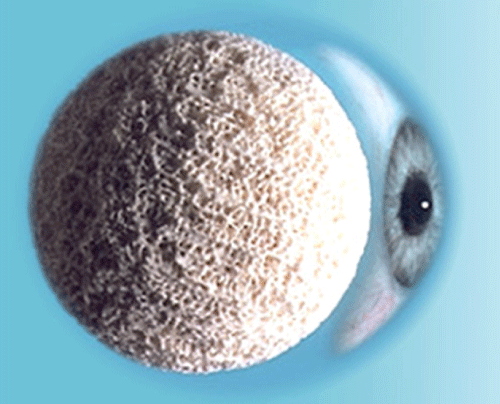
.
Figure 6: Porous spherical implant made of bioceramic (Photo courtesy Dr Patrick R. Boulos, eye surgeon).
View Figure 6
External prosthesis and iris
After the stable growth of tissue around the ocular implant, an imprint is taken of the cavity with the help of a mold in which alginate or quick seizing silicone is injected in order to define the shape of the future prosthesis, as seen in figure 8. The material used can also be a very resistant acrylic resin, also used in dentistry. It is to be noted that every ocularist uses their own specific recipe for fabrication, but they are all similar [9,23].
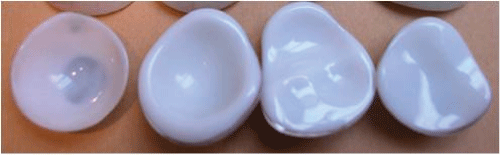
.
Figure 7: The four models of corresponding prostheses to figures 4-5 (Photo courtesy Jean-François Durette, ocularist).
View Figure 7
The imprint of the ocular cavity is used to make a wax model. In using this model, we can determine the position of the iris, the external curvature as well as the maximum surface of the eye that is exposed when open. On a "button", shown in figure 9A, the size of the pupil is first measured. The iris is hand-painted, aided by the direct observation of the patient's non-affected eye. As shown in figure 9B, the wax model is used to prepare the plaster mold, which, once hardened, will be filled with acrylic plastic to cover the prosthesis.
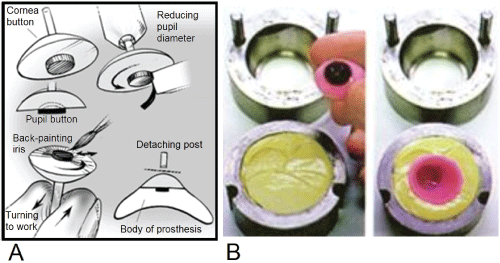
.
Figure 9: A) Fabrication of the iris; B) Creating the mold. Adapted from Hughes [24].
View Figure 9
The composition of this acrylic plastic that envelops the prosthesis is a mix of polymethyl methacrylate (PMMA) and methyl methacrylate (MMA), monomer of PMMA. The liquid MMA is mixed with the clear PMMA in a glass vase with the proportion of mass PMMA: 3.5 for MMA: 1. The acrylic preparation is occasionally mixed so that it becomes homogenous and then is left to rest for 20 to 30 minutes (it is faster at higher temperatures) until it reaches a pasty consistency. The wax that is currently surrounding the prosthesis is removed and the prosthesis is coated in this PMMA/MMA paste.
The porous nature of the prosthesis can provoke an unwanted coloration or bacterial infiltration, affecting its biocompatibility. To eliminate this porosity, the prosthesis is then cooked at 120°C under a pressure of 300 kPa for an hour in the device shown in figure 10.
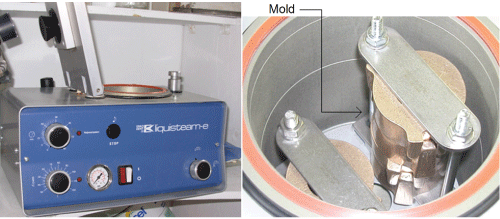
.
Figure 10: Equipment for the pressure-cooking of the prosthesis. Adapted from Lapointe [25].
View Figure 10
A touch of color is added to the prosthesis and blood vessels are drawn to add a natural appearance. The prosthesis is then given a transparent protective coating that is then polished to have a completely smooth surface that also provides the illusion of wetness. The prosthesis is hence complete and a perfect replica of the ocular cavity. In most of the cases, it is then comfortable and will stay in place.
Summary of Constraints Limiting the Choice of Technologies in Creating Dynamic Pupils
Limited space
Contrary to popular belief, ocular prostheses are not balls but half-shells. The thickness of this shell, where the device must be inserted, is between 1.5 and 15 mm. Additionally, recent prostheses have a tendency to be very thin, less than 4 mm thick.
Rough fabrication process
In order to make the prosthesis biocompatible, an essential step of the fabrication is the pressure-cooking, 120°C and at a pressure of 300 kPa for an hour.
Autonomy and self-powering of the device
In addition to the extremely limited workable space to insert a battery, the masse of the battery itself would provoke the eyelid to sag over the years. The use of ambient lighting as a source of energy to power the device becomes then primordial. Moreover, there must be no action required by the user in order for the pupil to vary in dimension. The main objective is that the users would entirely forget that they have an ocular prosthesis.
Suggested Technologies for Ocular Prosthesis with Dynamic Pupils
A magnetic interchangeable pupil
This innovation from LaFuente [26] is very simple and, to our knowledge, is the only one that has been commercially available. Figure 11 presents its functionality. A canal (F) is made in the ocular prosthesis (A). The background image is an iris (B) with a small pupil (C). A large pupil (D) with a magnet (E) can be moved with the help of another magnet along the canal. When it is darker or brighter, the patient can use a pocket magnet to manipulate the pupil. It must be noted that there are only two possible pupil dimensions. Moreover, since the patient is obligated to manually change his pupil size, it does not fulfill the main objective for dynamic pupil prostheses, allowing the patient to forget the loss of the eye (the devices autonomy). It is important however to highlight the fact that this device requires no battery.
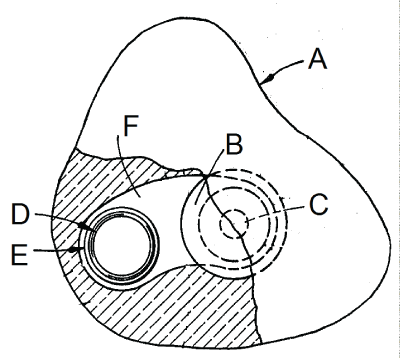
.
Figure 11: Ocular prosthesis (A) with an interchangeable pupil. B: Background iris with small pupil (C). Large magnetized (E) pupil (D) which goes along the integrated canal (F). Adapted from LaFuente [26].
View Figure 11
A polarized pupil that dilates with polarized glasses
The proposed technology by Young [27] is also very simple. As indicated in figure 12, the eye prosthesis has a polarized disk inserted between a small pupil and the image of the iris painted behind it. When the patient wears a pair of glasses with polarized lenses perpendicular to the polarization of the disk in the prosthesis, the pupil appears larger. Polarized lenses are visibly similar to sunglasses and must be worn when it is dark, in order to increase the diameter of the pupil. It can then appear strange that a person would be wearing sunglasses at night. Moreover, since one of the lenses is polarized, the other lens must be darker in order to appear similar, which will affect the patient's night vision. Let us note that this invention has the advantage of requiring no battery and the disadvantage of once again, providing only two possible sizes for the pupil.
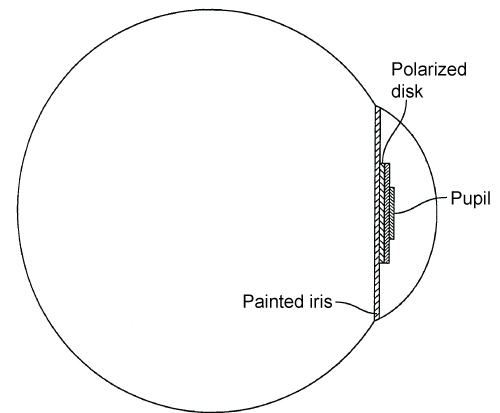
.
Figure 12: Sectional view of the polarized disk inserted in the prosthetic eye. Adapted from Young [27].
View Figure 12
The photochromic pupil
This invention by Friel [28] is the only one that meets both the requirement of no necessary battery while also providing more than two possible pupil sizes. Figure 13 presents its system. The principal is based on photochromic layers that go from transparent to opaque with the increase in luminosity. When it is dark, the photochromic layers are transparent and the large pupil is visible. When there is no more light, the photochromic layer behind becomes colored and the pupil becomes smaller. When the luminosity is intense, the two photochromic layers are colored and only the small pupil in front is visibly black. Meanwhile, it would be very difficult to recreate the image of the iris with photochromic materials. Since one material creates one color, a precisely composed matrix would have to be created from many different photochromic materials in order to recreate a realistic iris, which would be very complex. Additionally, most of these photochromic materials are activated under UV rays, so the device would only truly work outdoors.
Dynamic iris using a light source
Schleipman, et al. [29] propose an iris that changes the pupil diameter by using a light source. Figure 14 presents the device's components. Under the transparent section, in the middle of the image of the iris, is a small solar cell that detects light intensity. When the luminosity is high, a light source placed under the iris is completely illuminated. The image of the iris possesses many little holes to let this light through. When it is darker, the ring of light under the iris is extinguished, giving the pupil a larger appearance. The light source with many luminous rings is controlled by a battery powered electronic circuit.
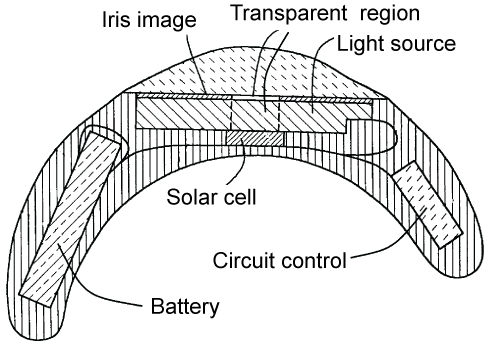
.
Figure 14: A dynamic iris with a light source. Adapted from Schleipman, et al. [29].
View Figure 14
Device with a display simulated live iris
The device developed by Wehbe [30] is presented in figure 15. First of all, the light intensity is measured with the help of a photodiode. A microcontroller then converts the analog signal provided by the photodiode into a digital 8-bit signal representing the luminosity, comparing this numerical value to the values input in its memory which finally, controls the display that simulates the human iris. This display is composed of light-emitting diodes (LED). The display and the electronic circuit require batteries.
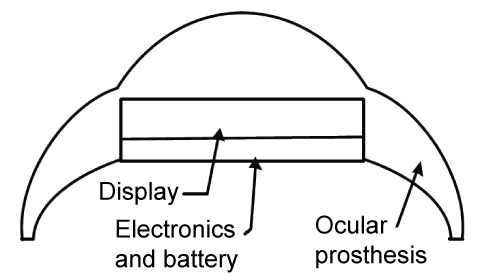
.
Figure 15: Device with a display simulating a live iris. Adapted from Wehbe [30].
View Figure 15
Device with liquid crystal display and memory
The device proposed by Budman [31] is similar to that of Wehbe, but instead of using an LED display, Budman uses a liquid crystal display (LCD). Figure 16 presents the sectional view of an ocular prosthesis using this display. Many iris images with different pupil sizes are stored in the devices memory and are displayed on the LC screen according to the ambient lighting detected by the photodiode. It is to be noted that the photodiode is on the outside of the region of the iris and is then visible. This innovation has the advantage of being able to reproduce a perfect image of a healthy live iris, regardless of the pupil size. This device also requires a battery.
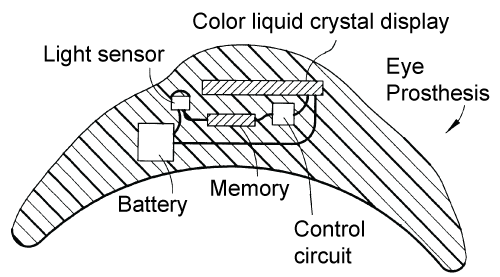
.
Figure 16: Dynamic iris with LC display and memory. Adapted from Budman and Stack [31].
View Figure 16
Simulating the iris with LC cells that block light
As seen in figure 17, the device proposed by Danz [32] uses a phototransistor that detects light intensity placed in the middle of the image of the iris. An LC cell shaped as a ring that can block and reveal the image of the iris, is placed in front of the iris. An electronic circuit, based on breakers, activates or not the LC cell depending on the ambient lighting. This circuit is also powered by a battery.
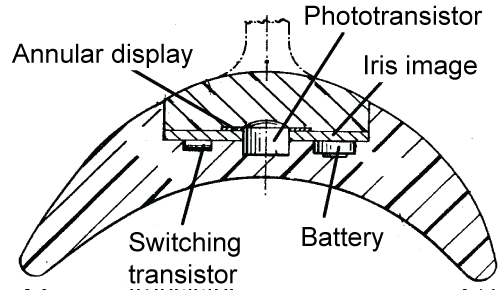
.
Figure 17: Dynamic iris using a LC cell ring that blocks out light. Adapted from Danz [32].
View Figure 17
Study in LCs for ocular prostheses
Leuschner's team studied a device that also uses liquid crystal technology [33,34]. As shown in figure 18, the image of the iris is blocked out by ring shaped pixels on an LC display. In their research, two types of LCs are studied: twisted nematic (TN) and polymer diluted LCs. Their studies with diluted LCs have concluded that this technology could not provide enough of a contrast to simulate an iris. On the other hand, the TN LCs, even if their results were inconclusive, lead one to believe that these LCs could be the solution to fixed pupils. They attribute this failure to the state of technology surrounding LCs, which, at the time, was not advanced enough. Their technology also requires a battery.
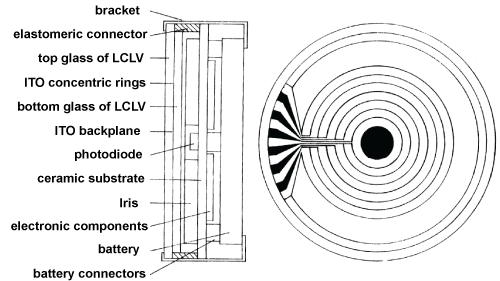
.
Figure 18: LC shutter. On the left: Components of the module. On the right: Transparent ITO Electrodes in concentrically shaped rings. Adapted from Leuschner [33].
View Figure 18
A New Ocular Prosthesis with a Dynamic Pupil
Here is a device recently developed by our group that satisfies the requirements previously discussed [36,36]. This device is composed of a liquid crystal cell (LCC) and a passive electronic circuit, autonomous and self-powered, using an appropriate solar cell (SC). The device survives the rough fabrication processes of the ocular prosthesis. For proper comprehension of this device's functionality, the principal functions of a liquid crystal cell are first presented. Then, the device design inside of the prosthesis is revealed. The electronic circuit as well as the utility of each of its components will then be explained. A prototype is then also presented, to conclude with a discussion of the remaining constraints that must be overcome in order to present a final wearable product.
Principle of the liquid crystal cell
A liquid crystal cell (LCC), as shown in figure 19, is composed of two glass plates (b) covered by thin layers of indium tin oxide (ITO) which serve the purpose of transparent electrodes (c). The LC (e) is inserted between the two glass plates. Two directional layers cover the electrodes to force a perpendicular orientation for the LCs on each side of the cell, giving the LCs a helical shape.
In figure 19A, the ambient light enters in the LCC from the right and is horizontally polarized by the polarizing filter (a). The light goes through the glass (b), the ITO electrode (c) and the orientation layer (g). Without applied potential, when the light goes through the LC, the polarization of the light obtains a 90° rotation, hence becoming vertically polarized due to the helical positioning and the birefringence of the LC. The sequence of layers forming the back of the LCC is identical to those of the entry. The vertically polarized light is at last blocked at the second polarizer that only allows horizontally polarized light to pass through. The image of the iris is glued to the back of the LCC. No light is reflected and the image of the iris appears as black.
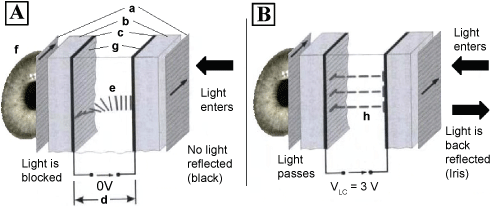
.
Figure 19: Principle of operation of the LCC. A: Without applied voltage, the image of the iris appears black; B: With applied voltage, the image of the iris is visible in reflection. Adapted from Lapointe, et al. [35].
View Figure 19
In figure 19B, under the effect of electrical fields created by the applied voltage of VLC, the positive dielectric anisotropy of the LCs (h) aligns with the direction of the electrical field and does not modify the polarization of the entering light, since the LCs are not birefringent in this direction. Indeed, the two components of the polarization see the same refractive index. The light then stays polarized horizontally and passes through the second polarizer. The image of the iris is reflected, passes through the LCC a second time and appears at the exit point. Using this concept, the passage of light can be controlled by means of the LCC.
Dynamic ocular prosthesis design
Figure 20A presents the general schematic of the ocular prosthesis with a pupil reacting to ambient lighting. First of all, on top, an LC cell with ring shaped pixels that can block out light can simulate a live pupil. Figure 20B shows the delimitation of electrodes in the LCC that are not visible to the naked eye.
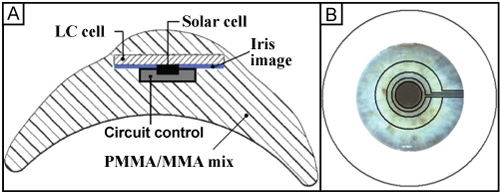
.
Figure 20: Schematic of the device. A: sectional view of the ocular prosthesis; B: Top view. Adapted from Lapointe, et al. [37].
View Figure 20
An image of an iris is glued under the LCC. The center of this image, of the same dimension as the minimum opening of the pupil (3 mm in diameter), is empty. A solar cell (SC), that reproduces effectively the colour of the pupil, is placed in that empty space. This SC in particular is described in section 6.4. The SC detects ambient lighting in order to control the diameter of the pupil, using a passive electronic circuit, described in the next section. In order to function, the LCC barely requires any current and only approximately 3 V. The current is determined by the leakage through the high resistivity LCC and the speed of switch-on. Since these two parameters can be extremely low, the power required is minimal and the LCC does not require any other source of energy other than that provided by the SC. It is hence important that the control mechanism is passive and that it consumes a minimum of energy. Using a novel passive circuit connected to the ring shaped electrodes, as well as the SC divided into many serial sections, each providing a voltage of 0.7 V [38], the adequate power can be easily achieved in order to operate the LCC, making the pupil dynamic and autonomous. A rechargeable battery is not desirable since it would limit the lifespan of the prosthesis (the battery would still require periodical replacement), would increase the cost and above all its mass, leading to possible eyelid sagging. This system resolves many important problems by reducing not only the cost and the need for long-term maintenance, but also the weight restrictions of the prosthesis.
The passive electrical circuit
Figure 21 presents the schematic of the electric components necessary for the dynamic pupil to function. This circuit is autonomous, self-powered and passively controls the diameter of the pupil. It is to be noted that the ITO electrodes are transparent. When it is dark, the power provided by the SC is weak and the LCC does not work. All of the ring shaped pixels are hence inactive, by using parallel polarizers, and appear as black. The light is blocked and the pupil seems dilated.
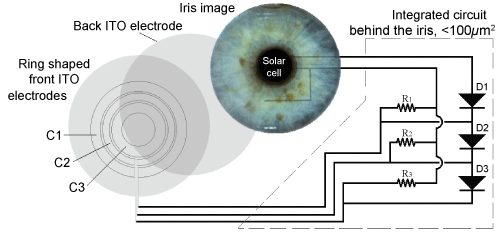
.
Figure 21: Schematic of the electrical circuit principal behind the dynamic pupil, using a passive voltage selector. Each ring shaped pixel acts as a capacitor. Adapted from Lapointe, et al. [35].
View Figure 21
Each ring shaped pixel acts like a capacitor (C1, C2 and C3). The largest electrode (C1) is connected in parallel with the resistor R1 and in series with the diode D1. The electrode in the middle (C2) is connected in parallel with the resistance R2 and in series with the diode D1 and D2. The smallest electrode (C3) is connected in parallel with the resistor R3 and in series with the diode D1, D2 and D3.
An LC pixel will let the light coming from the image of the iris pass through when the applied potential is of approximately 3V or more. A diode can cause the potential to fall by approximately VD = 0.5 V depending on the type of diode and the passing current. In order to power the largest pixel, the serially interconnected SCs must generate 3 V + 0.5 V = 3.5 V and the image of the iris is visible through this ring, which then makes the pupil smaller. With more light, the SC must generate 3 + 0.5 × n V in order to make the nth pixel function, leading to the pupil to appear smaller. Without the resistors connected in parallel, the current passing through the LCC is negligible since it is acting as a capacitor. With this current being close to zero, the fall of potential originating from the diode is also negligible and all of the pixels practically function under the same illumination. Meanwhile, with the resistors in place, the fall of potential in each diode becomes considerable and each pixel lights up sequentially with the increase in light intensity. Let us note that the first diode could be absent. This would increase the potential power channeled towards all pixels, but would eliminate a degree of freedom in the realistic simulation of the patient's pupil.
This circuit is very flexible and can be adjusted for most pupils that react differently under light. The number of interconnected SCs, the type of diode and the number of diodes can be changed in order to properly simulate the movement of the patient's pupil. The adjustment of the pupil is determined by the fall of potential in the diodes. Meanwhile, the resistors can be changed in order to precisely adjust the potential channeled to the electrodes. For example, if a patient reacts under weaker lighting, the resistor in parallel with the largest pixel can be increased.
The high voltage solar cell
Under the same illumination on its exposed surface, a solar cell (SC) generates a constant power of P = IV. As the system does not require considerable power, the SC can be modified in order to maximize potential V. Figure 22, on the left, shows the design of such a SC and, on the right, where it is located in the device.
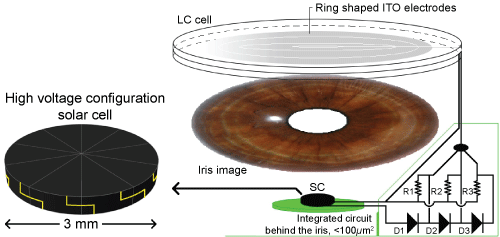
.
Figure 22: On the left: sectioned solar cell in order to maximise the voltage. On the right: location of installation of the SC that would recreate the smallest pupil size. Adapted from Lapointe, et al. [37].
View Figure 22
The SC is sectioned and by interconnecting each piece in series, the voltage is then multiplied. This concept is well-known and can generate an elevated voltage, more than the device needs with a SC of the same size as the minimum opening of the pupil (~3 mm in diameter) [38,39]. Using this concept, the device can be self-powered.
Each of the SC pieces must absorb the same quantity of light and hence must possess the same exposable surface. Indeed, since these SCs are in series, each one of them must be crossed by the same current. If there were SCs of different sizes, the current would be limited to the one generated by the smallest. In order to optimize the SC, a kind of pizza shape should be chosen.
Detailed calculation of the feasibility is demonstrated in previous work using standard SC [35]. Note that high efficiency black silicon SC have been recently demonstrated [40]. In addition to giving a better appearance to the pupil, black silicon SC would allow the use of lower purity of liquid crystal, which would reduce the cost of the final product.
The prototype
Here we show the LC artificial eye prototype, equipped with a pupil that changes dimension in accordance to ambient lighting. Figure 23 presents four different states that the pupils can have under different lighting conditions.
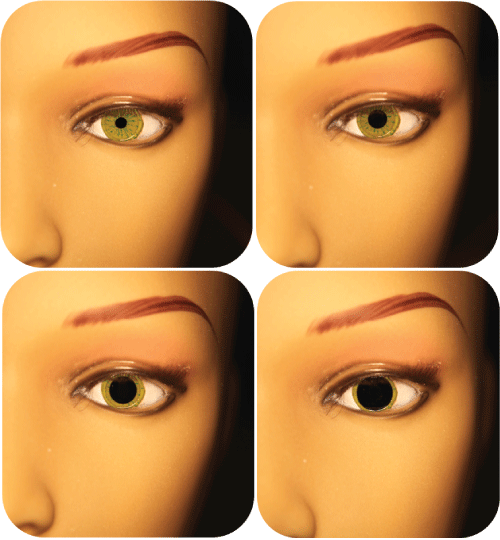
.
Figure 23: The working LC prototype with different pupil sizes, from A with high ambient light to D in the dark. (Photo courtesy JL).
View Figure 23
The LCC integrated in this prototype is presented alone in figure 24, on the left. The electric circuit, hidden behind the image of the iris, is presented alone in figure 24, on the right. The diameter of the circuit is inferior to 8 mm and hence can be hidden under the image of the iris that possesses a diameter of approximately 13 mm. This circuit is hand-made, passive and autonomous. It is self-powered using 9 square shaped SCs (3 × 3 mm) connected in series and placed outside of the prosthesis. This shows that 9 SC pieces is enough to generate the voltage needed. However, for the final device, the SC should follow the design shown in figure 22 and should use black silicon in order to fit the pupil color [40]. Although the circuit is ready to be integrated into the final device, it should be integrated in a thinner space in order to reduce the total thickness of the dynamic pupil device. The fabrication of the integrated circuit into the back of or around the SC would be something to consider.
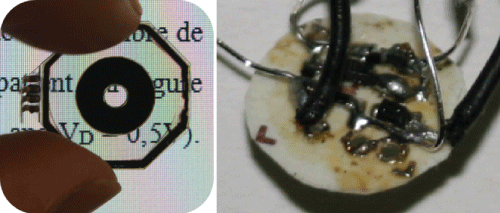
.
Figure 24: Left: liquid crystal cell in the state where it simulates a large pupil. Right: electrical circuit that can be hidden behind the iris. Adapted from Lapointe, et al. [36].
View Figure 24
Discussion and considerations concerning the final device
Of course, this first prototype is not ready to be commercialized. In addition to not being aesthetically pleasing, the device, including the SC and the circuit, must be optimized in order to perfectly follow the movement of the healthy eye. This discussion identifies certain trials, possible problems and solutions considered in order to create a device that could be functional and aesthetically acceptable. Furthermore, a new configuration of the electrodes, requiring no circuit and only a SC in order to power the device, is presented.
Dimension of the device: The thickness of the prosthesis varies between 1.5 to 15 mm, as is shown in figure 25. The integration of the device into thicker prostheses is not a problem, however, for those that are less than 4 mm thick, the integration becomes a more complex procedure. Furthermore, thinner prostheses are more and more commonly used. Indeed, porous spherical implants, as shown in figure 6, are increasingly popular in modern eye surgery and do not leave a substantial space for the prosthesis.
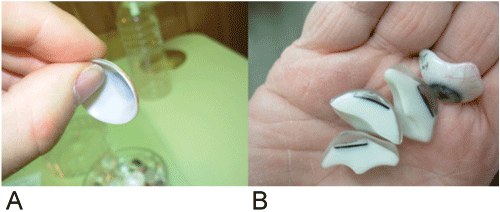
.
Figure 25: A) Very thin ocular prosthesis; B) Sections of ocular prostheses that are thicker. Adapted from Lapointe [25].
View Figure 25
It hence becomes primordial to find solutions in order to diminish the thickness of the device. The addition of thickness of the components of the prototype (LCC: 2.4 mm, circuit et CS: 1.5 mm) gives approximately 3.9 mm. The thickness of the LCC is limited by the rough conditions of the ocular prosthesis fabrication process. It is demonstrated that the square LCCs of 25 × 25 mm in dimension require glass plates with a minimum of 1 mm in thickness [35]. Meanwhile, the final LCC would be circular and 13 mm in diameter. Given that the force is proportional to the surface area, the thickness can be reduced to less than 0.25 times. The final LCC could have a thickness of approximately 0.5 mm. Since the thickness of the image of the iris is comparable to that of a sheet of paper, that the thickness of the SC is less than 0.5 mm and that the circuit could be built around the SC, the total thickness of the device could be less than 1 mm.
Even if we succeed in creating a device that is thinner than the smallest of prostheses, another problem comes into play: the curvature of the prosthesis. Indeed, as indicated in figure 26A, because of the rounded shape of the prosthesis, the superior corners of the device would surpass the edges of the prosthesis. This constraint could be overcome by using slightly curved glass plates, possessing exactly the same curvature as that of the prosthesis, as shown in figure 26B.
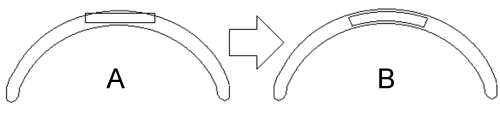
.
Figure 26: Limitations created by the curvature of thinner prostheses. Adapted from Lapointe [25].
View Figure 26
Electrode diameter: The best relation between the diameter of the human pupil and the intensity of incident light is [41]:
where D is the diameter of the pupil in millimetres and B is the luminance of the field of vision in millilamberts. In the case of a device massively produced, this equation could be used to determine the value of the internal and external diameters of each ring shaped pixel.
Meanwhile, certain factors like age, colour of the eye, any ametropia and pathological conditions also affect the size of the pupil [41]. In isolated cases where the variation of the pupil would not follow the norm, the patient's eye should be photographed under different light intensities and the LCC's electrodes could be engraved according to the needs of the patient.
It is important to also note that a real pupil is never perfectly round. The ITO electrodes could then be engraved in a way that is slightly imperfect in order to give the most natural looking result possible.
Alternative configuration of the electrodes: An interesting configuration to study is one where the distance between the two electrodes increases towards the center of the LCC. Figure 27 presents this configuration. The bottom electrode is simply a glass plate with an ITO layer. One of the advantages of this configuration is that no ITO ring needs to be engraved, allowing then to skip the steps of photolithography and the expensive cost of precision masks. The glass plate on top is made in two parts. For the bottom part (1), the surface that comes in contact with the LCs must be parallel to the lower glass plate in order to make the LCC working adequately. The thickness of the bottom part (1) must diminish with the radial. An ITO coating is then deposited. The part on top (2) must perfectly marry the surface of the bottom part (1).
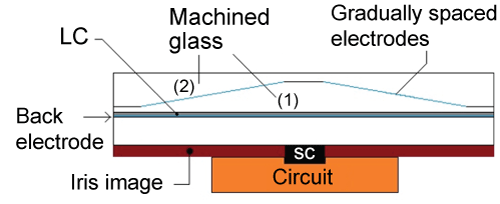
.
Figure 27: Electrode configuration where the distance between the two becomes greater at the center. Adapted from Lapointe [25].
View Figure 27
The functionality of this configuration is simple. The voltage necessary to activate a pixel is dependent on the distance between the electrodes (V = Emind, where Emin is the electric field necessary to align the LCs and d is the distance between the electrodes). A higher voltage is required (more light) in order to activate the region closer to the center of the LCC. Given that the distance between the electrodes d is gradual, the diameter of the pupil will also change gradually in accordance to variations in lighting.
At first glance, this configuration has the advantage of requiring no diode or resistor. The SC could be directly linked to the LCC. Only the variation in distance between the electrodes could be modified to adequately simulate the patient's pupil. The absence of an electronic circuit, in additional to the possibility of introducing the SC into the lower glass plate would allow to diminish the thickness of the device. Additionally, the more gradual variation in diameter of the pupil would probably lead to an even more natural effect.
However, the fabrication of the glass plates is more complex. In fact, this configuration of glass plate would probably be more vulnerable in the fabrication process of the ocular prosthesis. The interface between the two glass pieces (1) and (2) should be really solid to avoid the plate's flexure.
Conclusion
Given that the realistic appearance of ocular prostheses has been limited for several years by the immobile pupil, the conclusions of latest research have given back hope to the ambition of creating a "living" prosthesis. Indeed, it has been demonstrated that the practical solution of having a dynamic, autonomous and self-powered pupil is possible, while taking into account issues of size, fabrication process, weight, cost and mass production of the prostheses.
In order to conceive a functional and patient wearable prototype, many steps and constraints are still to be overcome. Namely, in order to respond to the demand of having ocular prostheses that are thinner and thinner, the methods to minimize thickness of the device must be considered. For example, integrating an electronic circuit on the edges of the SC and slightly curving the glass blades of the LCC are considerable options. Finally, considering that ocular prostheses with dynamic pupils could have a positive impact on the quality of life of patients, we all hope that this technology will be soon on the market.
Acknowledgments
We would like to thank Jean-François Durette, ocularist, and Dr Patrick R. Boulos, eye surgeon, for their technical explanations that helped us write this text. JL would like to thank his friend, Alexandre Laflamme, for kindly letting us photograph his eyes shown in figure 1. JL would also like to thank his friend, Audrey Meubus (http://audreymeubus.tumblr.com/), for providing language help. JL and RK acknowledge support from CIPI and the Canadian Govt.'s Canada Research Chairs program.
Competing Interests
None declared.
Ethical Approval
Not required.
References
-
Doshi P J, Aruna B (2005) Prosthetic management of patient with ocular defect. J Indian prosthodont soc 5: 37-38.
-
Artopoulou II, Montgomery PC, Wesley PJ, Lemon JC (2006) Digital imaging in the fabrication of ocular prostheses. J Prosthet Dent 95: 327-330.
-
Gordon B (1940) The ancient origins of artificial eyes, 3rd ed. Annuals of medical history: New York, USA.
-
Gray PH (1967) Radiography of ancient Egyptian mummies. Med Radiogr Photogr 43: 34-44.
-
Martin O, Clodius L (1979) The history of the artificial eye. Ann Plast Surg 3: 168-171.
-
Pare A (1561) Cimbrosie. Oruveres: Paris.
-
Dyer NA (1980) The artificial eye. Aust J Ophthalmol 8: 325-327.
-
Boissonneau M (1849) Yeux, artificial mobiles. Ritterich: Paris.
-
Raizada K, Rani D (2007) Ocular prosthesis. Cont Lens Anterior Eye 30: 152-162.
-
Mules P H (1885) Evisceration of globe with artificial vitreous. Trans Ophthalmol Soc UK 5: 200-206.
-
Helms H A, Zeiger H E J, Callahan A (1987) Complications following enucleation and implantation of multiple glass spheres in the orbit. Ophthalmic Plastic Reconstr. Surg 3: 87-89.
-
Hornblass A, Herschorn BJ (1985) Double sphere orbital implantation in enucleation and evisceration. Ophthal Plast Reconstr Surg 1: 65-68.
-
Tyers AG, Collin JR (1985) Baseball orbital implants: a review of 39 patients. Br J Ophthalmol 69: 438-442.
-
Johnson W J (1996) The perfect socket: the ocularist's view. In: S Bosniak (Ed.), Principles and practice of ophthalmic plastic and reconstructive surgery. Saunders: Philadelphia, 1127–1133
-
Perry AC (1990) Integrated orbital implants. Adv Ophthalmic Plast Reconstr Surg 8: 75-81.
-
Perry A C (1991) Advances in enucleation. Ophthalmol. Clin North Am 4: 173-182.
-
Karesh JW, Dresner SC (1994) High-density porous polyethylene (Medpor) as a successful anophthalmic socket implant. Ophthalmology 101: 1688-1695.
-
Moshfeghi DM, Moshfeghi AA, Finger PT (2000) Enucleation. Surv Ophthalmol 44: 277-301.
-
Rubin P A, Popham J K, Bilyk J R, Shore J W (1994) Comparison of fibrovascular ingrowth into hydroxyapatite and porous polyethylene orbital implants. Ophthalmic Plast Reconstr Surg 10: 96-103.
-
Song JS, Oh J, Baek SH (2006) A survey of satisfaction in anophthalmic patients wearing ocular prosthesis. Graefes Arch Clin Exp Ophthalmol 244: 330-335.
-
Durette J F (2005) The Durette Ocular Implants, Acrylic Revisited. J Ophthal Prosth 10: 7-18.
-
Custer PL, Kennedy RH, Woog JJ, Kaltreider SA, Meyer DR (2003) Orbital implants in enucleation surgery: a report by the American Academy of Ophthalmology. Ophthalmology 110: 2054-2061.
-
Bartlett SO, Moore DJ (1973) Ocular prosthesis: a physiologic system. J Prosthet Dent 29: 450-459.
-
Hughes M O (2015) Artificial eye clinic 2010; Consulted on December 7, 2015:
-
Lapointe J (2010) Prothèse d'oeil photonique. ProQuest Dissertations and Theses. Thesis (M.Sc.A.). Ecole Polytechnique, Montreal (Canada), Publication Number: AAT MR70479.
-
LaFuente H (1982) US Patent No. 4,332,039. Washington, D.C.: U. S. P. a. T. Office.
-
Young S R (2007) US Patent No. US 7,311 397 B1. Washington, D.C.: U. S. P. a. T. Office.
-
Friel T P (1991) US Patent No. 5,061,279. Washington, D.C.: U. S. P. a. T. Office.
-
Schleipman F, Schleipman R, Sleet D P V, Duncunson P A (2000) US Patent No. 6,139,577. Washington, D.C.: U. S. P. a. T. Office.
-
Wehbe M (2009) Dynamic pupil reacting to incident light dedicated to ocular implants. M.Sc.A. École Polytechnique de Montréal, Qc., Canada.
-
Budman M, Stack J R (2003) US Patent No. US 6,576,013 B1. Washington, D.C.: U. S. P. a. T. Office.
-
Danz W R (1981) 4,272,910. Washington, D.C.: U. S. P. a. T. Office.
-
Leuschner F W (1992) Light-controlled pupil size for ocular prosthesis. Proceedings of ophthalmic technologies II 1644: 320-325.
-
Seekolaq D L, Leuschner F W (1995) Use of liquid crystal display technology in ocular prosthesis. Liquid crystal materials, devices and displays 2408: 100-106.
-
Lapointe J, Durette JF, Harhira A, Shaat A, Boulos PR, et al. (2010) A 'living' prosthetic iris. Eye (Lond) 24: 1716-1723.
-
Lapointe J, Kashyap R, Boulos P R (2010) US Patent No. P1217USPR.
-
Lapointe J, Harhira A, Durette J F, Beaulieu S, Shaat A, et al. (2011) An ocular prosthesis which reacts to light. Proc. SPIE 7885, Ophthalmic Technologies XXI, 788512.
-
Green M A, Wenham S R, Zhao J (1993) Progress in high efficiency silicon cell and module research. Conference Record of the IEEE Photovoltaic Specialists Conference 8-13.
-
Lee JB, Chen Z, Allen M G A (1995) A Miniaturized High-Voltage Solar Cell Array as an Electrostatic MEMS Power Supply. J Microelectromechanical Syst 4: 102–108.
-
Oh J, Yuan HC, Branz HM (2012) An 18.2%-efficient black-silicon solar cell achieved through control of carrier recombination in nanostructures. Nat Nanotechnol 7: 743-748.
-
S. G. de Groot, J. W. Gebhard (1952) Pupil size as determined by adapting luminance. J Opt Soc Am 42: 492-495.
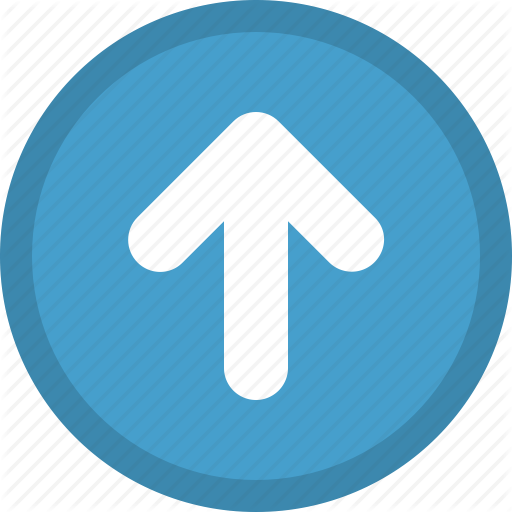