International Journal of Stem cell Research and Therapy
A Conceptual Integration of Extra-, Intra- and Gap Junctional- Intercellular Communication in the Evolution of Multi-cellularity and Stem Cells: How Disrupted Cell-Cell Communication during Development can Affect Diseases later in Life
James E Trosko*
Department of Pediatrics/Human Development, College of Human Medicine, Michigan State University, Michigan, USA
*Corresponding author: James E Trosko, Ph.D., Professor Emeritus, Department of Pediatrics/Human Development, College of Human Medicine, Michigan State University, East Lansing, Michigan 48824, USA, E-mail: james.Trosko@hc.msu.edu
Int J Stem Cell Res Ther, IJSCRT-3-021, (Volume 3, Issue 1), Review Article; ISSN: 2469-570X
Received: December 10, 2015 | Accepted: January 26, 2016 | Published: January 29, 2016
Citation: Trosko JE (2016) A Conceptual Integration of Extra-, Intra- and Gap Junctional-Intercellular Communication in the Evolution of Multi-cellularity and Stem Cells: How Disrupted Cell-Cell Communication During Development can Affect Diseases later in Life. Int J Stem Cell Res Ther 3:021. 10.23937/2469-570X/1410021
Copyright: © 2016 Trosko JE. This is an open-access article distributed under the terms of the Creative Commons Attribution License, which permits unrestricted use, distribution, and reproduction in any medium, provided the original author and source are credited.
Abstract
An attempt will be made to provide a short conceptual review to integrate, from an evolutionary perspective, how the emergence of gap junctional intercellular communication helped to bring about multi-cellularity and new adaptive phenotypes. This new fundamental biological function of the metazoans was needed to provide homeostatic control of new cellular functions of an interacting society of different cell types existing in a 3-dimensional unit. Changing paleo-physics- and -chemistry of the earth led to single celled organisms that metabolized sugar via glycolysis and survived via symmetrical cell division and occasional mutations. With the appearance of oxygen-producing phytoplanktons, the single cell organism, the mitochondrion, symbiotically- fused with a primitive cell to form the first multi-cellular organism, which could metabolize glucose via oxidative phosphorylation. The new society of adherent cells developed new strategies for adaptive survival. New genes and phenotypes included: (a) growth control, (b) differentiation, (c) programmed cell death; (d) senescence; (e) regulation of gene expression-"epigenesis"; (f) germline and somatic stem cells; (g) asymmetrical cell division; and (h) anoxic stem cell niches. The evolutionary development of the normal human organism, starting from a single "toti-potent" stem cell to the mature, reproductive and self-aware being, consisting of over 100 trillion cells, of which 200 different cell types and having three major functional cells- (organ-specific stem cells; their progenitor derivative cells; and their differentiated daughters), could only come about by a delicate homeostatic integrated feedback system of extra-, intra- and gap junctional inter-cellular communication (GJIC). Since GJIC occurs in all organs, any disruption of the three forms of cell communication mechanisms by genetic or epigenetic factors, particularly during embryonic, fetal and neonatal periods, could lead to alteration of risks to diseases later in life (i.e., the Barker hypothesis). Chronic disruption of these signaling mechanisms in the adult organs could also lead to several kinds of chronic, stem cell-based diseases, diabetes, cancer, atherogenesis and premature aging.
Keywords
Extra-cellular communication, Intra-cellular signaling, Gap junctions, Connexins, Stem Cells, Evolution, Mitochondrion, Barker Hypothesis
Introduction
The goal of this Short Review is to provide a conceptual explanation of how evolutionary forces in the changing physical environment of Earth, ultimately, gave rise to germinal and somatic stem cells and to an integrated process of extra-, intra-, and gap junctional inter-cellular communication mechanisms that led to the emergence of (a) the self-aware- conscious mind from the biological brain; and (b) either normal healthy human development or of multiple acute and chronic diseases. It is designed to cover multiple areas to which the evolution of the family of the gap junction genes, the "connexins", can contribute to the understanding of the delicate homeostatic regulation of cell proliferation, differentiation, apoptosis and senescence in the normal development of the human being and how disruption of cell-cell communication by epigenetic means, especially during in utero development, can lead to stem cell-based diseases. This conceptual review is a natural application of the quote by Theodosius Dobzhansky, when he said: "Nothing in biology makes sense except in the light of evolution [1]".
Background
In the early stages of the creation of Earth, its environment (atmosphere, water, temperature, radiation levels) were inhospitable to life forms. Eventually, the stage was set for the first life forms to emerge in an anoxic environment. These single cell organisms emerged to generate energy for life and reproduction by metabolizing glucose via a DNA-coded protein –enzyme mechanism, called glycolysis. It was a sufficient, but inefficient, mechanism to produce ATP, which, when metabolized, release the energy for life's processes. Only after the evolutionary appearance of phytoplanktons, which could capture energy from the sun to produce glucose and release oxygen, did the earth's environment change. Ironically, this new oxygenated environment became toxic to the anaerobic bacteria that now had to seek non-oxygenated niches [2-4]. While still in a scientific "black box", a new microorganism, the mitochondrion, appeared, which could metabolize glucose via "oxidative phosphorylation" to produce, more efficiently, ATP (hence more energy) [5]. What is not known is how this new energy- proficient biological energy factory, somehow, became symbiotically- associated with another cell type to become the parent for the first multi-cell, metazoan organism.
New genes and phenotypes needed for the evolutionary transition from single cell organisms to the multi-cell, metazoan organism
What was the evolutionary "quantum leap" of this first multi-cell metazoan organism? One needs to understand how the singled cell organism survived inevitable environmental change. Since most life forms depend on the DNA molecule as its "blue print" for evolutionary transmission of molecular and biochemical instructions for both individual survival and for the survival of the species, it must protect their DNA from either too many irreversible changes and too few (or both the individual and its species would die). In terms of these early single cell organisms, it had to select genes that could protect and repair damage to their genes so that not too many mutations would occur or, on the other hand, that these genes could not be too perfect so that no mutations would occur. The primary manner, how the singled cell organism survived the inevitable environmental change, was by "symmetrical" cell division. That is, when a mother cell divided, both daughters would be similar to the mother. However, in a large population of these single cell organisms, there could and would be a few daughters who had inherited a mutation in one of their gene repertoire. Out of a large population in a changing environment, most individual organisms that maintained their mother's gene repertoire would not survive. Only those that had inherited a mutation in a gene, which could cope with the new environment, would survive to maintain the species with a slight genomic change. In other words, survival of singled cell organisms was basically dependent on symmetrical cell proliferation.
The new adaptive strategy that evolution selected with the oxygenation of the earth's environment was a society of adhering cells that had other means to survive the changing environment. This came about when molecules, such as collagen, which could only be synthesized with the presence of oxygen, provided an "extracellular glue" so that cells could form an adherent society of cells in 3-dimensional structures [6,7]. However, whereas the single cells could communicate with each other via simple secreted molecules "quorum sensing" [8], these new 3-dimensional society of cells had to acquire genes for new forms of communication within and between these early metazoan cells. It appears that a family of genes, the connexins [9,10], which code for a membrane bound protein channel, that allows ions and small molecules to be transferred directly from one cell to its contiguous neighbor [11-15].These "multi-cell" metazoans acquired new phenotypes from new genes that allowed them to (a) control their growth; (b) divide both asymmetrically and symmetrically to form new specialized cells ( germinal stem cells; somatic stem cells, transit amplifying or somatic cells and highly specialized differentiated cells, such as eye, muscle, heart, neuronal, gonad, pancreas, etc. cells); (c) die by apoptosis ( or controlled cell death or suicide); (d) senesce; and (e) adaptively respond, via epigenetic mechanisms, if the cell is differentiated (e.g. to produce insulin in the beta cell of the pancreas when presented with glucose). The appearance of the connexin genes and functioning gap junctions also allowed for the development of the left-right bilateral symmetry [16].
Unique properties of stem cells: the ability to divide either by symmetrical or asymmetrical means
It is important to understand the concepts just mentioned, e.g. (a) symmetric and asymmetrical cell division; and (b) stem cells and somatic cells. The stem cell is capable of dividing either by symmetrical division (when the mother cell divides to produce two similar daughters) or asymmetrical division (when the mother cell divides to produce one daughter similar to itself- "unlimited self- renewal" and the other daughter that is free to have finite ability to proliferate and to terminally differentiate into a highly specialized cell, such as a neuron). One hypothesis for this ability to switch between these two means of cell proliferation could be determined by factors signaling the division plane of the stem cell in the niche, which might or might not regulate the expression of the connexin gene [17]. They are, for all intensive purposes, "immortal" cells until they are induced to "mortalize" by apoptosis, by senescence or by terminally differentiation. The other cell type, the somatic or transit amplifying cells, can only divide symmetrically for a finite amount of time before it dies by apoptosis, senesces or terminally differentiates.
In the human being, a single "toti-potent" stem cell (the fertilized egg) divides to form "pluri-potent" stem cells, which through the process of development gives rise to "multi-potent" stem cells to form different organ systems and to give rise to uni-potent stem cells that form unique lineages of highly specialized cells, such as a hepatocyte or a red blood cell.
Distinct modes of cell communication between single cell organisms and metazoans
Two important observations have to be highlighted here. First, think of single cell organisms dividing in the primitive earth. As long as they have nutrients and the right exposure to radiation/temperature and other physical & chemical (pH; chemical toxicants) restraining factors, they will proliferate until those limiting factors inhibit their ability to divide.
A population of free-standing single cell organisms can and do communicate with each other via primitive forms of hormones or growth factors ("Quorum-sensing"). That is to say, secreted factors from individual single cell organisms can communicate with other members to control certain cellular behaviors. They normally proliferate as long as nutrients are abundant and negative signaling molecules are absent. In other words, cybernetic regulation does occur when nutrients become depleted. During log phase growth, these single cell organisms act as metaphorical cancer cells.
With the abundance of oxygen, the molecular family of collagen type molecules, allowed cells to stick together to form society of adherent cells that could now exist in 2- and 3-dimensions [6]. While single cell organisms can and do exist in 2-dimensional biofilms [18], the ability to form a 3-dimensional and communicating society of different functioning cell types, required not only extra-cellular communication of secreted-type molecules over space and distances but that of direct transfer of ions and small regulatory molecules between adhering cells, namely via gap junctions. This new architecture of a society of cells required gap junctional intercellular communication a new means to communicate in order to maintain homeostatic control of the functions of cells, namely , growth control; differentiation, apoptosis, epigenetic regulation of specific genes of the total genome and senescence. The formation of an adhering society of cells must control their ability to proliferate because, if they do not, they are, indeed, a cancer. That is, cancer cells are those that lose control growth, do not terminally-differentiate, do not perform apoptosis normally, have abnormal gene expression and are characterized as being "immortal" [19]. In addition to the known functions of the gap junctions, the emerging roles of hemichannels is starting to provide newer insights of their roles in the communication of cell functions in the 3-dimensional society of cells [20].
To put this observation in context of this "Short Review", several genes appeared during the evolutionary transition [21] from an anaerobic environment to an oxygenated environment to allow this adherent population to control growth, to differentiate and to perform apoptosis during critical periods of development [22]. This family of highly conserved genes contains the connexin genes. These genes code for the connexin proteins that self assemble into a hexameric hemichannel ("connexon"), and when transferred to the cell membrane, it unites with the opposing "connexon" to form a channel that allows ions and small molecular weight molecules to transfer, freely, from one cytoplasm directly to the other cell cytoplasm, without having to transverse the cell membrane, the inter- cellular space, and to re-enter the neighboring membrane to get into the cytoplasm [11]. A floating plaque on the cell membrane of these conjoined connexons forms the gap junctions, which can now help to synchronize metabolic and electrotonic functions of groups of cells. This allows cardiomyocytes to electrotonically contract in synchrony or allows synchronization, metabolically, of all the hepatocytes of the liver to all express the genes to detoxify potential toxicants in the liver. From the transcription of the connexin genes, their translation into proteins, their self-organization into hemichannels, the translocation to the membrane, the assembly into a functional gap junction by fusion with opposing connexon, the organization into floating plagues and any posttranslational modification of the individual connexins proteins of the gap junctions, there are multiple points of regulation. The channel size, determined by the specific connexon components of the gap junctions, determine the specific molecules that might traverse the gap junction channel. This selective transfer of ions/small molecules, including micro-RNA's [23], can help to determine the gene expression of the communicating cells and their phenotypes and functions.
It turns out the toti-potent stem cell (the fertilized cell) does not express its connexin genes and, therefore, has no functional gap junctions [22]. As the fertilized egg starts to proliferate, at an early stage of the embryonic blastomere, some connexin genes are expressed and the cells start to differentiate [24]. These differentiated cells, in turn, secrete extracellular molecules (e.g. extracellular matrix molecules; cell adhesion molecules, hormones, growth factors, etc.) [25-28], that, in turn, could induce other cells to up-regulate different connexins to cause additional cells to differentiate into other cell types. Therefore, in the carefully controlled chaos of communication mechanisms of the developing embryo/fetus, extra-cellular-secreted molecules (hormones, growth factors, cytokines, neurotransmitters), bind to expressed receptors on sequestered differentiated cells to trigger "intra-cellular signals" [29]. These specific "intra-cellular" signaling pathways (a) turn different subsets of the total genome off or on, as well existing biochemical structures and functions, in each cell type, and (b) increase or decrease connexin gene expression (epigenetically) or function to proliferate, differentiate or go through apoptosis [30,31]. Consequently, in the development of the embryo, fetus and neonate, it is critical that a delicate regulation of gene expression, cell proliferation, differentiation and apoptosis must occur or else, development could be disrupted that could lead to embryonic/fetal death, birth defects, and modulation of diseases later in life could occur (The "Barker hypothesis" [32]). One potential mechanism that could explain how exposure to endogenous or exogenous agents during pregnancy might increase or decrease the risk to diseases later in life is that these agents could increase or decrease organ-specific adult stem cells [33-35], that could be the target cells for stem cell-based diseases, such as diabetes, cancer or atherosclerosis [36,37]. While this concept of the fundamental roles that gap junctional intercellular communication plays in the normal physiological functions of all organs of the metazoans, including the human being, this concept is still not generally recognized as key factor in the fields of toxicology, cancer, neurosciences, nor in the emerging field of stem cell regenerative therapy, as it should. However, it is a fact that these gap junctions exist in all organs and the gap junctional intercellular communication process, which, homeostatically, regulates cell proliferation, differentiation and apoptosis can be modulated by all kinds of physical, chemical and biological agents, as well as by behaviors, such as smoking; excessive calories and cultural conditions (changing diets; lack of exercise) [38,39]. With the explosion of experiments linking alterations of the gut microbiome to various chronic diseases of the human [40,41], and with the known association of gut bacteria, Heliobacter pylori, and stem cells in the stomach [42,43], as well as various secreted inflammatory factors from various populations of the microbiome that could modulate gap junctions in the gut cells [44], one could reasonable speculated that these factors could stimulate normal or "initiated" adult gut stem cells to bring about chronic inflammatory bowel diseases or intestinal cancers.
Stem Cell Characteristics
During the evolutionary transition from the anoxic environment to the oxygenated environment, the germinal and somatic stem cells were formed and had to be sequestered in an anoxic microenvironment (their niche) in the multi-cellular metazoan to allow it to remain quiescent for most its time [45], except during organism growth, wound healing, germ cell formation, and cell replacement. The reason the stem cell and its low oxygen-microenvironment, its niche, were needed is because, if this founding metazoan cell was subject to differentiation or "mortality", it would not have survived. Consequently, metazoans had to develop a delicate homeostatic relationship between the primitive-type of stem cell (germinal and somatic) for the species survival and individual's survival. This convergence of selected genes during the evolution of the metazoan led to these new phenotypes of (a) cell division regulation ("contact-inhibition"), (b) differentiation of different cell types, (c) apoptosis of cells during development and tissue repair, (d) stem cells and their niches, (e) epigenetic regulation of selective gene expression and (f) senescence. The stem cell is characterized by metabolism of glucose via glycolysis, since it lacks the number of mitochondria found in its progenitors and differentiated daughters [46-50]. Upon exogenous signals, including exposure to oxygen [51-54], the cells can divide by either symmetrical division to increase the stem cell number or by asymmetrical cell division to form one daughter to retain "stemness" and one daughter that is destined to form a lineage-dependent differentiated cells.
Therefore, these stem cells must have a low oxygenated niche to retain their stemness abilities later in life for wound repair, growth and tissue regeneration. The transit amplifying or progenitor cells, which will eventually die, are needed only for growth of organs and tissue replacement. The differentiated cells, which gave up their "immortality" to perform high energy- requiring functions, acquired more mitochondria to generate their need for more energy by oxidative phosphorylation. They can usually give up their highly differentiated functions because, as long as the adult organ –specific stem cells survive, they can be replaced.
There are major implications of this view of the evolutionary emergence of the mitochondrion, the stem cells, the gap junction gene family, and symmetrical and asymmetrical cell division. One reason of both the anoxic niche environment (needed for stemness with few mitochondria and glycolysis of glucose for energy) and oxygenated environments for oxidative phosphorylation by somatic cells (needed for high energy metabolic production for differentiate functions) is that a highly, cybernetic feedback of specific signals from extra-, intra- and gap junctional inter-cellular communication must control mutually exclusive cellular choices the cell makes.
Difference between normal and stem cells, cancer stem cells and single cell organisms
One of the major hypotheses of the origin of cancer cells, in particular, "cancer stem cells" or "cancer initiating cells" (the cells in a tumor that sustain the growth of tumors) [55] is that they seem to originate from normal adult organ-specific stem cells, rather than from "de-differentiating" or by being "re-programmed" [19,50,56-58]. Both the normal stem cells and the cancer cells metabolize glucose via glycolysis, in a manner similar to single cell organisms [59].
Since cancer cells do not have growth control, do not terminally differentiate, nor do they apoptosis, senesce, or divide asymmetrically, and since they lack either the expression of the gap junction genes or function of the gap junctions, cancer cells might be viewed as retro-evolutionary throw-back to the single cell organisms that proliferate only symmetrically and in an uncontrolled fashion [50,58].
One example of how abnormal dysfunction of an organ-specific adult stem cell might lead to cancer can be found in the association of various viruses, such as hepatitis, SV40, human papilloma viruses, and various human cancers [60]. Some of these oncogenic viruses have been referred to as "immortalizing" viruses. That is, the prevailing interpretation of the role of these viruses and associated "oncogenes" is that, when integrated into differentiated or "mortal" cell types, they are able to "de-differentiate" or "re-program" these "mortal cells", so that embryonic genes are re-expressed and they become "immortalized". Once "immortalized", they can survive longer to accrue other genetic and epigenetic alterations to acquire those "hallmarks of cancer" [61,62]. The SV40 Large T antigen can inactivate the p53 and RB gene products, preventing terminal differentiation [63]. However, an alternative interpretation of this prevailing cancer paradigm can be, and has been made, namely, these viruses do not "immortalize" or "re-program" differentiated cells, but rather, when a population of cells, containing a few adult stem cells, many progenitor and terminally-differentiated cells, in vitro or in vivo, are exposed to these viruses, only the adult stem cells (which is naturally immortal until induced to terminally differentiate or become "mortal"), containing the virus, will be blocked from becoming "mortal". In other words, oncogenic viruses or their "oncogenes" only affect the normal adult tissue specific stem cells (cervix, breast, liver, etc). These cells are not yet neoplastically transformed, but can survive long enough to accrue those other changes to become invasive and metastatic [64].
Of course not all cancers need viruses to become cancerous. Various types of mutations, inherited, such as in the inherited ultraviolet light skin cancer syndrome, xeroderma pigmentosum [65], which lacks the ability to repair UV-induced DNA lesions [66] and are hyper-producing somatic mutations via "errors of DNA repair" [67,68]. However, given recent controversy on the potential role mutations formed in proliferating stem cells [69-80], what has been ignored is the fact that mutations can be formed in dividing adult stem cells via "errors in DNA replication", such as in the cancer prone syndrome, Bloom syndrome, which forms mutations without DNA lesions [81]. Therefore, cancers could arise from stem cells, either from mutations caused by "errors of DNA repair" or "errors of DNA replication" that could block these stem cells from terminal differentiation, allowing them to live longer to accrue other needed changes to become malignant.
The alteration of the quality & quantity of stem cells leads to the yin & yang nature of cancer and aging
Aging, itself, as recently demonstrated by the Hutchinson Gilford-Progeria human premature aging syndrome, could be due to the genetic loss of stem cells [82], or in the case of "normal" aging, by environmental agents inducing their loss. Indeed, as in the linkage of cancer and aging, sun-exposed skin can cause premature aging of the skin and skin cancer by either killing or mutating skin stem cells, respectively. Smoking can induce lung stem cell death or premature differentiation, leading to emphysema or asthma or the promotion of initiated or premalignant lung stem cells, leading to lung cancer. Finally, excessive alcohol can kill liver stem cells leading to cirrhosis or promoting premalignant liver stem cells to liver cancer.
Finally, the brain, which has multiple communicating regions, which, in turn, control different operational functions by the extra-, intra- and gap junctional inter-cellular mechanisms of glial, oligodendrocytes, and neurons [83] can be altered during early development to give rise, not only to unconscious control of body functions, but, also, to altered awareness of becoming self-aware ("As a result of a thousand million years of evolution, the universe is becoming conscious of itself, able to understand something of its past history and its possible future".-Julian Huxley). By diurnal control of the integrated cell-cell communication process via the feedback of light ( possibly by the alteration in melatonin [84]), daily metabolic breakdown molecules, exposures to drugs, dietary factors, stress, etc., this homeostatic regulation of these three forms of cell communication can explain both normal development and health, but also many diverse diseases associated within all organs.
Conclusions
The development of a normal, healthy, self conscious human being starts from a single fertilized egg dependents on the delicate concatenation of a hierarchical and cybernetic interaction of three integrated cell communication mechanisms, coded by many genes that are influenced by both endogenous and exogenous factors (extra-cellular communication) that triggers intra-cellular communication within stem cells, transit amplifying cells and terminally differentiated cells, which, in turn, modulates gap junctional inter-cellular communication to initiate mutually exclusive cell behaviors. The complexity of this process, especially during early development, renders disruption of development very likely since the organism has but one chance to "get it right". In the adult, disruption of this integrated communication process in highly stable organs, usually by massive cell killing, can lead to serious life threatening diseases [85]. On the other hand, chronic non-cell killing exposures of disruption of cell-cell communication in particular organs can lead to much organ-specific pathology. The major implications of this perspective of the fundamental role of cell –cell communication in the homeostatic regulation of cell proliferation, differentiation and apoptosis, particularly acutely, during in utero development, and chronically, during neonatal, adolescent, maturation and geriatric phases of life, is that alteration of stem cell numbers and quality of stem cells could affect stem cell-based diseases.
References
-
Dobzhansky T (1975) Nothing in biology makes sense except in the light of evolution. Am Biol Teach 35:125-129.
-
Nursall JR (1959) Oxygen as prerequisite to the origin of metazoan. Nature 183: 1170-1172.
-
Saul JM, and Schwartz L (2007) Cancer as a consequence of the rising level of oxygen in the Precambrian. Lethaia 40: 211-220.
-
Saul JM (2008) Did detoxification processes cause complex life to emerge? Lethaia 42: 179-184.
-
Schmidt CW (2010) Mito-conundrum: Unraveling environmental effects on mitochondria. Environ. Health Perspect. 118: A292-297.
-
Saul JM (2014) A Geologist Speculates on Gemstones, Origin of Gas and Oil, Moonlike Impact Scars on the Earth, The Emergence of Animals and Cancer. Les 3 Colonnes, Paris.
-
Doljanski F (2004) The sculpturing role of fibroblast-like cells in morphogenesis. Perspect Biol Med 47: 339-356.
-
Miller MB, Bassler BL (2001) Quorum sensing in bacteria. Annu Rev Microbiol 55: 165-199.
-
Willecke K, Eiberger J, Degen J, Eckardt D, Romualdi A, et al. (2002) Structural and functional diversity of connexin genes in the mouse and human genome. Biol Chem 383: 725-737.
-
Cruciani V, Mikalsen SO (2005) The connexin gene family in mammals. Biol Chem 386: 325-332.
-
Bruzzone R, White TW, Paul DL (1996) Connections with connexins: the molecular basis of direct intercellular signaling. Eur J Biochem 238: 1-27.
-
Goldberg GS, Moreno AP, Lampe PD (2002) Gap junctions between cells expressing connexin 43 or 32 show inverse permselectivity to adenosine and ATP. J Biol Chem 277: 36725-36730.
-
Evans WH, Martin PE (2002) Gap junctions: structure and function (Review). Mol Membr Biol 19: 121-136.
-
Mehta PP (2007) Introduction: a tribute to cell-to-cell channels. J Membr Biol 217: 5-12.
-
Yamasaki H, Naus CC (1996) Role of connexin genes in growth control. Carcinogenesis 17: 1199-1213.
-
Chuang CF, Vanhoven MK, Fetter RD, Verselis VK, Bargmann CI (2007) An innexin-dependent cell network establishes left-right neuronal asymmetry in C. elegans. Cell 129: 787-799.
-
Trosko JE, Chang CC (2010) Factors to consider in the use of stem cells for pharmaceutic drug development and for chemical safety assessment. Toxicology 270: 18-34.
-
Greenberg EP (2003) Bacterial communication and group behavior. J Clin Invest 112: 1288-1290.
-
Trosko JE (2009) Review paper: cancer stem cells and cancer nonstem cells: from adult stem cells or from reprogramming of differentiated somatic cells. Vet Pathol 46: 176-193.
-
Batra N, Kar R, Jiang JX (2012) Gap junctions and hemichannels in signal transmission, function and development of bone. Biochim Biophys Acta 1818: 1909-1918.
-
Revel JP (1988) The oldest multicellular animal and its junctions. In: Hertzberg EL, Johnson R, (Eds.), Gap Junction (pp 135-149) NY: Alan Liss, Inc.
-
Trosko JE, Chang CC, Wilson MR, Upham B, Hayashi T, et al. (2000) Gap junctions and the regulation of cellular functions of stem cells during development and differentiation. Methods 20: 245-264.
-
Lim PK, Bliss SA, Patel SA, Taborga M, Meneka A Dave, et al. (2011) Gap Junction-Mediated Import of MicroRNA from Bone Marrow Stromal Cells Can Elicit Cell Cycle Quiescence in Breast Cancer Cells. Cancer Res 71: 1550-1560.
-
Lo CW1 (1996) The role of gap junction membrane channels in development. J Bioenerg Biomembr 28: 379-385.
-
Horiguchi K, Kouki T, Fujiwara K, Kikuchi M, Yashiro T (2011) The extracellular matrix component laminin promotes gap junction formation in the rat anterior pituitary gland. J Endocrinol 208: 225-232.
-
Meyer RA, Laird DW, Revel JP, Johnson RG (1992) Inhibition of gap junction and adherens junction assembly by connexin and A-CAM antibodies. J Cell Biol 119: 179-189.
-
Puri CP, Garfield RE (1982) Changes in hormone levels and gap junctions in the rat uterus during pregnancy and parturition. Biol Reprod 27: 967-975.
-
Trosko JE, Chang CC, Madhukar BV, Klaunig JE (1990) Chemical, Oncogene and Growth Factor Inhibition of Gap Junctional Intercellular Communication: An Integrative Hypothesis of Carcinogenesis. Pathobiology 58: 265-278.
-
Même W, Calvo CF, Froger N, Ezan P, Amigou E, et al. (2006) Proinflammatory cytokines released from microglia inhibit gap junctions in astrocytes: potentiation by beta-amyloid. FASEB J 20: 494-496.
-
Upham BL, Trosko JE (2009) Carcinogenic tumor promotion, induced oxidative stress signaling, modulated gap junction function and altered gene expression. Antioxidants & Redox Signaling 11: 297-308.
-
Wilson MR, Close TW, Trosko JE (2000) Cell population dynamics (apoptosis, mitosis, and cell-cell communication) during disruption of homeostasis. Experimental Cell Res 254: 257-268.
-
Barker DJ (2004) The developmental origins of adult disease. J Am Coll Nutr 23: 588S-595S.
-
Trosko JE (2008) Role of diet and nutrition on the alteration of the quality and quantity of stem cells in human aging and the diseases of aging. Curr Pharm Des 14: 2707- 2718.
-
Trosko JE, Suzuki K (2009) Adult stem cells, the Barker Hypothesis , epigenetic events and low level radiation effects. In: Radiatiation Health Risk Sciences. Springer Publisher 216-226.
-
Trosko JE (2011) The gap junction as a "Biological Rosetta Stone": Implications of evolution, stem cells to homeostatic regulation of health and disease in the Barker Hypothesis. J Cell Commun & Signal 5: 53-66.
-
Trosko JE, Chang CC, and Upham BL (2002) Modulation of gap junctional communication by 'epigenetic toxicants': A shared mechanism in teratogenesis, carcinogenesis, atherogenesis, immunomodulation, reproductive-and neuro-toxicities. In: Biomarkers of Environmentally Associated Disease. S.H. Wilson and W.A. Suk, eds., Lewis Publishers 445-454.
-
Trosko JE (2001) Gap junctional intercellular communication: A ‘Biological Rosetta Stone' concept for understanding ‘Epigenetic' toxicology. J. Toxicol. Pub. Health 17: 1-9.
-
Trosko JE (2014) Global Health Crisis Caused by the Collision of Biological and Cultural Evolution: Pre-Natal Influences on Acute and Chronic Diseases in Later Life. Planet@Risk 2: 271-280.
-
Trosko JE (2015) Global Bioethical Prevention of the Collision of Biological and Cultural Evolution on Miserable Human Survival. Sociology Study. 5: 295-231
-
Lukens JR, Gurung P, Vogel P, Johnson GR, Carter RA, et al. (2014) Dietary modulation of the microbiome affects autoinflammatory disease. Nature 516: 246-249.
-
Ohman L, Tornblom H, Simrén M (2015) Crosstalk at the mucosal border: importance of the gut microenvironment in IBS. Nat Rev Gastroenterol Hepatol 12: 36-49.
-
Bessède E, Dubus P, Mégraud F, Varon C (2015) Helicobacter pylori infection and stem cells at the origin of gastric cancer. Oncogene 34: 2547-2555.
-
Bessède E, Staedel C, Acuña Amador LA, Nguyen PH, Chambonnier L, et al. (2014) Helicobacter pylori generates cells with cancer stem cell properties via epithelial-mesenchymal transition-like changes. Oncogene 33: 4123-4131.
-
Mine T, Kushima R, Fujita T (1997) Relationship between healing of acetic acid-induced chronic gastric ulcer and connexin. J Clin Gastroenterol 25 Suppl 1: S111-115.
-
Marshman E, Booth C, Potten CS (2002) The intestinal epithelial stem cell. Bioessays 24: 91-98.
-
Nesti C, Pasquali L, Vaglini F, Siciliano G, Murri L (2007) The role of mitochondria in stem cell biology. Biosci Rep 27: 165-171.
-
Armstrong L, Tilgner K, Saretzki G, Atkinson SP, Stojkovic M, et al. (2010) Human induced pluripotent stem cell lines show stress defense mechanisms and mitochondrial regulation similar to those of human embryonic stem cells. Stem Cells 28: 661-673.
-
Chen CT, Shih YR, Kuo TK, Lee OK, Wei YH (2008) Coordinated changes of mitochondrial biogenesis and antioxidant enzymes during osteogenic differentiation of human mesenchymal stem cells. Stem Cells 26: 960-968.
-
Prigione A, Fauler B, Lurz R, Lehrach H, Adjaye J (2010) The senescence-related mitochondrial/oxidative stress pathway is repressed in human induced pluripotent stem cells. Stem Cells 28: 721-733.
-
Trosko JE (2014) Induction of iPS cells and of cancer stem cells: the stem cell or reprogramming hypothesis of cancer? Anat Rec (Hoboken) 297: 161-173.
-
Csete M (2005) Oxygen in the cultivation of stem cells. Ann N Y Acad Sci 1049: 1-8.
-
Pervaiz S, Taneja R, Ghaffari S (2009) Oxidative stress regulation of stem and progenitor cells. Antioxid Redox Signal 11: 2777-2789.
-
Mohyeldin A, Garzón-Muvdi T, Quiñones-Hinojosa A (2010) Oxygen in stem cell biology: a critical component of the stem cell niche. Cell Stem Cell 7: 150-161.
-
Brigelius-Flohé R, Flohé L (2011) Basic principles and emerging concepts in the redox control of transcription factors. Antioxid Redox Signal 15: 2335-2381.
-
Atena M, Reza AM, Meehran (2014) A review on the biology of cancer stem cells. Stem Cell Discovery 4: 83-89.
-
Tai MH, Chang CC, Kiupel M, Webster JD, Olson LK, et al. (2005) Oct4 expression in adult human stem cells: evidence in support of the stem cell theory of carcinogenesis. Carcinogenesis 26: 495-502.
-
Trosko JE (2008) Human adult stem cells as the target cells for the initiation of carcinogenesis and for the generation of "cancer stem cells". Int J Stem Cells 1: 8-26.
-
Trosko JE, Kang KS (2012) Evolution of energy metabolism, stem cells and cancer stem cells: how the warburg and barker hypotheses might be linked. Int J Stem Cells 5: 39-56.
-
Warburg O (1956) On respiratory impairment in cancer cells. Science 124: 269-270.
-
zur Hausen H (1991) Viruses in human cancers. Science 254: 1167-1173.
-
Hanahan D, Weinberg RA (2000) The hallmarks of cancer. Cell 100: 57-70.
-
Hanahan D, Weinberg RA (2011) Hallmarks of cancer: the next generation. Cell 144: 646-674.
-
Ahuja D, Sáenz-Robles MT, Pipas JM (2005) SV40 large T antigen targets multiple cellular pathways to elicit cellular transformation. Oncogene 24: 7729-7745.
-
Kang KS, Trosko JE (2011) Stem cells in toxicology: fundamental biology and practical considerations. Toxicol Sci 120 Suppl 1: S269-289.
-
Cleaver JE (1978) Xeroderma pigmentosum: genetic and environmental influences in skin carcinogenesis. Int J Dermatol 17: 435-444.
-
Cleaver JE, Trosko JE (1970) Absence of excision of ultraviolet-induced cyclobutane dimers in xeroderma pigmentosum. Photochem Photobiol 11: 547-550.
-
Maher VM, McCormick JJ (1976) Effect of DNA repair on the cytotoxicity and mutagenicity of UV irradiation and of chemical carcinogens in normal and xeroderma pigmentosum cells. In: J.M. Yuhas, R.W. Tennant and J.D. Regan (Eds.), Biology of Radiation Carcinogenesis; New York: Raven Press 129-145.
-
Glover TW, Chang CC, Trosko JE, Li SS (1979) Ultraviolet light induction of diphtheria toxin-resistant mutants of normal and xeroderma pigmentosum human fibroblasts. Proc Natl Acad Sci US 76: 3982-3986.
-
Couzin-Frankel J (2015) Biomedicine. The bad luck of cancer. Science 347: 12.
-
Couzin-Frankel J (2015) Science Communication. Backlash greets 'bad luck' cancer study and coverage. Science 347: 224.
-
Wodarz D, Zauber AG (2015) Risk factors and random chances. Nature 517: 563-564.
-
Ashford NA, Bauman P, Brown HS, Clapp RW, Finkel AM, et al. (2015) Cancer risk: role of environment. Science 347: 727.
-
Potter JD, Prentice RL (2015) Cancer risk: tumors excluded. Science 347: 727.
-
Wild C, Brennan P, Plummer M, Bray F, Straif K, et al. (2015) Cancer risk: role of chance overstated. Science 347: 728.
-
Gotay C, Dummer T, Spinelli J (2015) Cancer risk: prevention is crucial. Science 347: 728.
-
Song M, Giovannucci EL (2015) Cancer risk: many factors contribute. Science 347: 728-729.
-
Tomasetti C, Vogelstein B (2015) Cancer risk: role of environmentâ€"response. Science 347: 729-731.
-
Tomasetti C, Vogelstein B (2015) Variation in cancer risk among tissues can be explained by the number of stem cell division. Science 347: 78-8.
-
Ledford H (2015) Cancer studies clash over mechanisms of malignancy. Nature 528: 317.
-
Song Wu, Scott Powers, Wei Zhu, Yusuf A (2015) HannunSubstantial contribution of extrinsic risk factors to cancer development. Nature 529: 43–47.
-
Warren S, Schultz RA, Chang CC, Wade MH, Trosko JE (1981) Elevated spontaneous mutation rate in Bloom syndrome fibroblasts. Proc. Natl. Acad. Science USA, 78, 3133-3137.
-
Rosengardten Y, McKenna T, Grochová D, Eriksson M (2011) Stem cell depletion in Hutchinson-Gilford progeria syndrome. Aging Cell 10: 1011-1020.
-
Spray DC, Rolf Dermietzel R (2013) Gap Junctions in the Nervous System. Springer Science & Business Media, Berlin.
-
Blackman CF, Andrews PW, Ubeda A, Wang X, House DE, et al. (2001) Physiological levels of melatonin enhance gap junction communication in primary cultures of mouse hepatocytes. Cell Biol Toxicol 17: 1-9.
-
Trosko JE, Upham, BL (2010) A paradigm shift is required for risk assessment of potential Human health after exposure to low level chemical exposures: A response to the Toxicity Testing in the 21st Century Report. Internatl J Toxicology 29: 344-357.
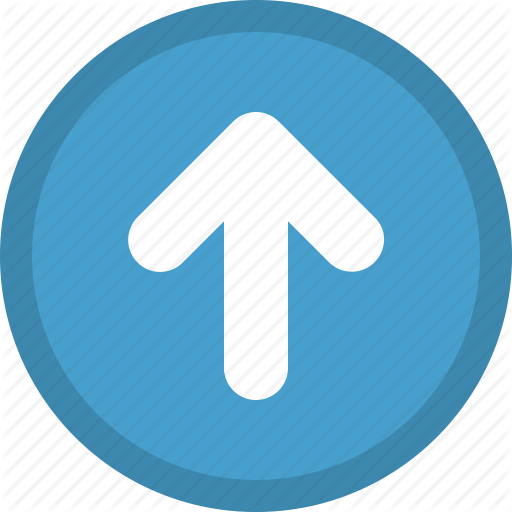