International Journal of Virology and AIDS
Exposure to HIV-1 Altered CCR7-Mediated Migration of Monocytes: Regulation by PGE2
Sandra C Côté, Stamatoula Pasvanis and Nancy Dumais*
Departement de Biologie, Faculte des Sciences, Universite de Sherbrooke, Sherbrooke (QC), Canada
*Corresponding author: Nancy Dumais, Faculté des Sciences, Université de Sherbrooke, Sherbrooke (QC), J1K 2R1, Canada, Tel: +1-819-821-8000, Fax: +1-819-821-8049, E-mail: nancy.dumais@usherbrooke.ca
Int J Virol AIDS, IJVA-2-011, (Volume 2, Issue 2), Research Article; ISSN: 2469-567X
Received: August 25, 2015 | Accepted: September 30, 2015 | Published: October 04, 2015
Citation: Cote SC, Pasvanis S, Dumais N (2015) Exposure to HIV-1 Altered CCR7-Mediated Migration of Monocytes: Regulation by PGE2. Int J Virol AIDS 2:011. 10.23937/2469-567X/1510011
Copyright: © 2015 Côté SC, et al. This is an open-access article distributed under the terms of the Creative Commons Attribution License, which permits unrestricted use, distribution, and reproduction in any medium, provided the original author and source are credited.
Abstract
Monocytes play critical roles in human immunodeficiency virus type-1 (HIV-1) pathogenesis. During HIV-1 infection, proinflammatory molecules such as prostaglandin E2 (PGE2) are observed at elevated levels in infected individuals. Mono-Mac-1 cells as well as freshly isolated monocytes were exposed to pseudotyped, R5 or dual tropic HIV-1 particles. CCR7 expression was determined by FACS analysis while chemotaxis assays were performed to show CCR7 functionality. We demonstrated that PGE2 enhanced CCR7 surface expression on monocytes. However, monocytes exposition to R5 or dual tropic HIV-1 particles did not highly modulate surface expression of CCR7. On the other hand, monocytes exposition to HIV-1 impaired the CCR7 dependent migratory capacity to CCL19. The addition of PGE2 to HIV-1-exposed monocytes restored the CCR7 dependent migration to levels similar to PGE2 treated unexposed monocytes. Monocytes acquired a functional responsiveness to CCL19 after exposure to PGE2 only when gp120 is expressed on viral particles. Finally, HIV-1-exposed monocytes that migrated in a Transwell system efficiently transmitted the infection to sensible cells. Collectively, we show that PGE2 is essential for the CCR7-dependent migration of monocytes exposed to HIV-1.
Keywords
Monocytes, HIV, CCR7-dependent migration, PGE2
Background
Transmission and pathogenesis of the Human Immunodeficiency Virus type-1 (HIV-1) are interconnected during all stages of the disease with cells of the monocyte/macrophage lineage [1-3]. Monocytes are constantly exposed or are targets of HIV-1 and their migratory behaviors are implicated in the dissemination of the infection throughout the host [4-8]. Monocytes arising from quiescent and infected cells of the myeloid precursor lineage in bone marrow have been demonstrated as a source of residual HIV DNA [1,7,9-12]. Despite treatment with antiretroviral therapies, monocytes can harbor latent HIV-1 proviral DNA during all stages of the disease forming a long-lived reservoir for the virus [4] and appropriate stimulation and/or differentiation can reactivate productive replication [6,13,14]. Infected circulating monocytes can migrate to peripheral tissues such as the brain [13,15], lung [16], lymphatic system [17], bone marrow [9], and kidney [18] but the implicated mechanism is poorly understood.
The chemokine receptor CCR7 plays an important role in the migration behavior of immune cells. CCL19 and CCL21 are the natural ligands of CCR7, which is not only expressed on DCs [19] but also on T and B cells [20-22] as well as on monocytes [23]. Mice deficient in CCL19, CCL21 or CCR7 demonstrate defective DC trafficking and altered immune response [24,25]. In human monocytes and DCs, prostaglandin E2 (PGE2), a pleiotropic immunomodulatory molecule, exerts multiple effects on both CCR7 expression level and functionality [23,26,27].
Since it has been shown that monocytes are actively implicated in HIV-1 pathogenesis and serum level of PGE2 is a hallmark of HIV progression [28-30], we asked whether PGE2 modulates CCR7 expression and functionality on HIV exposed monocytes. We investigated the cell surface expression of CCR7 in monocytes exposed to R5 or dual tropic strains of HIV-1. In parallel, chemotaxis assays were performed to establish the influence of HIV-1 exposition on CCR7 functionality. We also sought to determine whether the presence of PGE2 could modify the migratory capacity of monocytes exposed to HIV-1. Our results provide evidence that PGE2 restores functional CCR7 expression on HIV-exposed human monocytes allowing their migration toward the CCR7 ligand CCL19. Expression of CCR7 on HIV-exposed monocytes in response to PGE2 may have significant implications for HIV-1 dissemination to secondary lymphoid organs and/or peripheral tissues.
Methods
Ethics statement
The study was approved by the local ethics committee (Comité d'éthique de la Recherche sur l'Humain, Centre Hospitalier Universitaire de Sherbrooke, Université de Sherbrooke (04-116-R5)) and written consent was obtained from all participants.
Cells
Mono-Mac-1 cells, an acute peripheral monoblastic leukemia derived cell line (German Collection of Microorganisms and Cell Cultures, Braunschweig, Germany) was cultured in RPMI 1640 (Wisent, St-Bruno, QC, Canada) supplemented with 10% heat-inactivated fetal bovine serum (FBS) (Wisent), non essential amino acids (NEAA) (Wisent), 1 mM sodium pyruvate (Wisent), 100 I.U. penicillin, and 100 μg/ml streptomycin (Wisent). Human embryonic kidney 293T cells (American Type Culture Collection (ATCC) Manassas, VA) and the TZM-bl HIV-1 indicator cell line (NIH AIDS Research and Reference Reagent Program) were cultured in Dulbecco's modified Eagle's medium (DMEM) (Wisent) supplemented with 10% heat-inactivated FBS, NEAA, 1 mM sodium pyruvate, 100 I.U. penicillin, and 100 μg/ml streptomycin. TZM-bl is an indicator cell line derived from HeLa cells, which express the HIV-1 receptors CD4, CXCR4, and CCR5 and carry a stably integrated luciferase reporter gene under the control of HIV-1 long terminal repeat (LTR) [31]. Human monocytes were isolated from healthy donors' blood using Lymphocyte Separation Media as recommended by manufacturer (Wisent) and left to adhere on culture plates for 1 hour at 37℃ in RPMI 1640 supplemented as described above. Suspension cells were removed by two washes with phosphate buffered saline (PBS) and adherent cells were detached using Cell Dissociation Media (Wisent), washed with PBS, and used immediately.
Production of viral stocks
Pseudotyped HIV-1 particles were obtained by co-transfection of pNL4-3-Luc+E-R+ with pcDNA-I/Amp-based expression vectors coding HIV-1 ADA (R5), JR-FL (R5) envelope proteins or pHCMV-G vesicular stomatitis virus envelope glycoprotein G (VSV-G) under the control of the human cytomegalovirus promoter. The infectious molecular clones pcDNA1/89.6 and pNL4-3balenv were also used. All those plasmids were kindly provided by Dr. M. J. Tremblay (Centre de Recherche en Infectiologie, CHUL, Québec, Canada). The virus-containing supernatants were collected 48 hours after transfections with FuGENE® 6 (Roche Applied Science, Laval, QC), filtered through a 0.45-μm cellulose acetate, and normalized for virion content using a p24 ELISA as described previously [32].
Exposition of monocytes to HIV-1
Freshly isolated blood monocytes and Mono-Mac-1 were exposed to HIV-1 strains using 100 ng p24 per million cells for 1 hour at 37℃, washed with PBS, and seeded at 1 × 106 cells per ml in RPMI 1640 supplemented. Cells were then left untreated or treated with 1 μM PGE2 (Sigma Aldrich, Oakville, ON, Canada).
Chemotaxis assays
Chemotaxis of HIV-1 exposed blood isolated monocytes and Mono-Mac-1 was measured by migration through a polycarbonate filter with 5- or 8-μm pore size respectively, in 24-wells transwell chambers (Millipore, Nepean, ON, Canada). The lower chamber contained 500 μl of a 300 ng/ml dilution of CCL19 in medium without FBS (R&D Systems, Minneapolis, MN, USA), or medium alone as a spontaneous migration control, and the upper chamber contained 5 × 105 cells in 100 μl of medium. Blood isolated monocytes containing chambers were incubated for 2 hours at 37℃ and Mono-Mac-1 containing chambers were incubated for 4 hours at 37℃. A 100 μl aliquot of the cells that migrated to the bottom chamber were used for the infectivity assay or mixed with 200 μl of a 2% paraformaldehyde solution and counted using BD FACSCalibur flow cytometer (BD Biosciences) by acquiring events for a fixed period of 60 seconds using CellQuest software (BD Biosciences). The percentage of migrated cells was calculated as follow: the number of migrated cells in response to media only was subtracted to the number of migrated cells to CCL19 and this number was reported on the total input of cells.
Infectivity assays
Reporter TZM-bl cells were seeded at 104 cells/well in 96-well plates 12 hours before the assay. A 100-μl aliquot for the bottom well of the migration assay (see above) was added to the TZM-bl cells in triplicate. Cells were co-cultured for 2 hours and then the media was replaced with fresh complete media. The cells were incubated for an additional 48 hours at 37℃ and analyzed for luciferase activity [33].
Flow cytometry
Monocytes were collected and washed twice with ice-cold PBS. The Fc receptors were blocked using 1 μg purified IgG (Santa Cruz Biotechnology, Santa Cruz, CA, USA) for 10 minutes on ice. Mono-Mac-1 cells were stained with a phycoerythrin (PE)-conjugated anti-CCR7 antibody (R&D Systems). Blood isolated monocytes were double-stained with PE-conjugated anti-CCR7 and a Peridinin chlorophyll protein complex (PerCP)-conjugated anti-CD14 antibody (BD Biosciences, Mississauga, ON, Canada) or were incubated with corresponding isotypes as negative controls. Cells were then washed twice with ice-cold PBS supplemented with 3% bovine serum albumin (BSA) and fixed for 30 minutes on ice in 2% paraformaldehyde. Fluorescence was read using a BD FACSCalibur flow cytometer (BD Biosciences) and results were analyzed using CellQuest software (BD Biosciences).
Statistical analysis
Each experiment was performed at least three times in triplicate. Statistically significant differences between experimental groups were evaluated using a paired t-test. Computations were carried out using GraphPad PRISM® version 5.0b statistical software.
Results
Surface expression of CCR7 on HIV-1 exposed human monocytes is modulated by PGE2
We initially characterized the surface expression of CCR7 on HIV-1 exposed monocytes without or with PGE2 treatment (Figure 1). Our results demonstrated that exposition to pseudotyped viruses did not considerably alter cell surface expression of CCR7 compared to mock-treated cells (Figure 1A). However the addition of PGE2 to HIV-1-exposed Mono-Mac-1 was associated with an increase of CCR7 expression compared to PGE2-treated uninfected cells. The number of CCR7+ cells increased of 20.5% in uninfected cells and 9.7% and 15.4% in cells exposed to NL4-3-Luc+E-R+/Adaenv and NL4-3-Luc+E-R+/JR-FLenv, respectively. Next, Mono-Mac-1 cells were exposed to replication competent viruses (NL4.3balenv and 89.6) and FACS analysis revealed that CCR7 expression was slightly affected by the viral exposition (Figure 1B), with uninfected cells being 54.5% CCR7+ and 61.7% and 59.0% for NL4.3balenv and 89.6, respectively. The addition of PGE2 to replication competent HIV-1-exposed Mono-Mac-1 cells also augmented CCR7 cell surface expression as observed with cells exposed to pseudotyped viruses. PGE2 treatment increased CCR7 expression of 25.0% in uninfected cells and 17.8% and 21.1% in cells exposed to NL4.3balenv and 89.6, respectively. Since, Mono-Mac-1 cells are an immortalized cell line whose growth properties have been altered by transformation, we repeated this series of experiments with freshly isolated blood monocytes from healthy donors. As above, we determined the extent to which HIV-1 exposure and PGE2 treatment affects CCR7 cell surface expression in freshly isolated blood monocytes (Figure 1C). In mock-treated cells, 8,94% of CD14+ cells expressed CCR7. Exposition to NL4.3balenv and 89.6 reduced CCR7 expression to 4,78% and 5,22%, respectively. Stimulation of uninfected blood monocytes with PGE2 increased CCR7 cell surface expression for uninfected cells (14,41%), as well as blood monocytes exposed to HIV-1 (NL4.3balenv: 12,65% and 89.6: 10,09%). This degree of increased CCR7 surface expression is functionally relevant since other reports have shown that similar percentages of CCR7 expression enhance cell migration in response to CCL19 in the presence of PGE2 [23,26,27]. Collectively, these data demonstrate that addition of PGE2 invariably increased cell surface expression of CCR7 in both Mono-Mac-1 cells and freshly isolated blood monocytes. In contrast, HIV-1 exposure did not significantly modulate the expression CCR7 on monocytes. Indeed in Mono-Mac-1, exposition to pseudotyped HIV-1 particles slightly decreased cell surface expression of CCR7 while CCR7 levels were unchanged in Mono-Mac-1 cells. In freshly isolated blood monocytes exposition to R5 or dual tropic HIV-1 particles did not significantly change CCR7 expression.
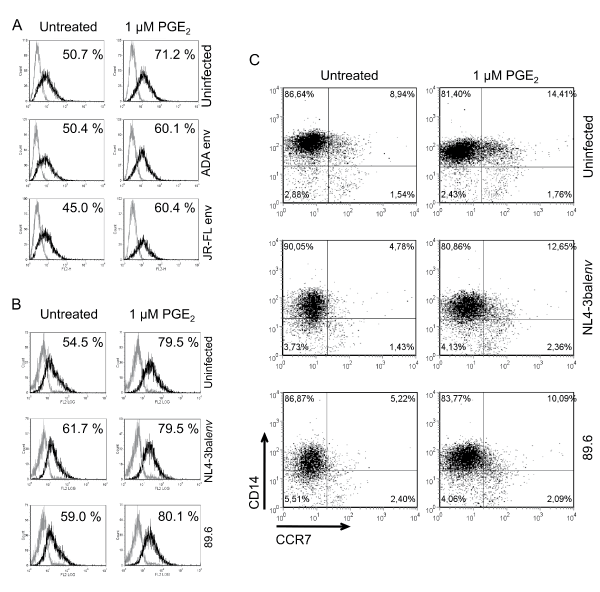
.
Figure 1: PGE2 enhances CCR7 surface expression on HIV-1 exposed monocytes
Mono-Mac-1 cells were exposed to the indicated pseudotyped (A) or replication competent (B) HIV-1 clones and left untreated or treated with 1 μM PGE2 for 24 hours. FACS analysis was performed on cells labeled by a CCR7-specific antibody (black line) or corresponding isotype control (gray line). Percentages of positive cells are indicated. Results represent 1 out of 5 independent experiments with similar results. (C) Blood isolated monocytes were exposed to the indicated HIV-1 replication competent clones and left untreated or treated with 1 μM PGE2 for 24 hours. Cells were double-stained for CD14 and CCR7 using specific antibodies and analyzed by flow cytometry. Quadrants were positioned based on corresponding isotypes. Results are from 1 of 4 different donors with similar responses.
View Figure 1
PGE2 enhanced CCR7-dependent migration to CCL19 of infected monocytes
We next assessed CCR7 function by measuring the migratory response of cells to the chemokine CCL19. The chemotaxis assays showed that PGE2 substantially increased Mono-Mac-1 cell migration in response to the CCR7 ligand CCL19 (Figure 2), as previously observed [23]. Incubation of Mono-Mac-1 cells with pseudotyped HIV-1 particles (NL4-3-Luc+E-R+/Adaenv or NL4-3-Luc+E-R+/JR-FLenv) resulted in specific migration of 6.9 ± 3.8% for NL4-3-Luc+E-R+/Adaenv and 5.9 ± 3.8 % for NL4-3-Luc+E-R+/JR-FLenv, which were similar to the migration behaviour of unexposed cells, 6.2 ± 3.5 % (Figure 2A). In contrast, exposition to fully infectious HIV-1, NL4.3balenv or 89.6, induced a significant augmentation in the percentage of migrating cells compared to the unexposed Mono-Mac-1 cell control (Figure 2B). Interestingly, PGE2 treatment of cells exposed to either pseudotyped or fully infectious viruses increased the percent of migrating cells to levels similar to unexposed Mono-Mac-1 cells (Figure 2A and Figure 2B). We demonstrated that the observed migration was CCR7 specific by showing a loss of migration upon blocking CCR7 (data not shown).
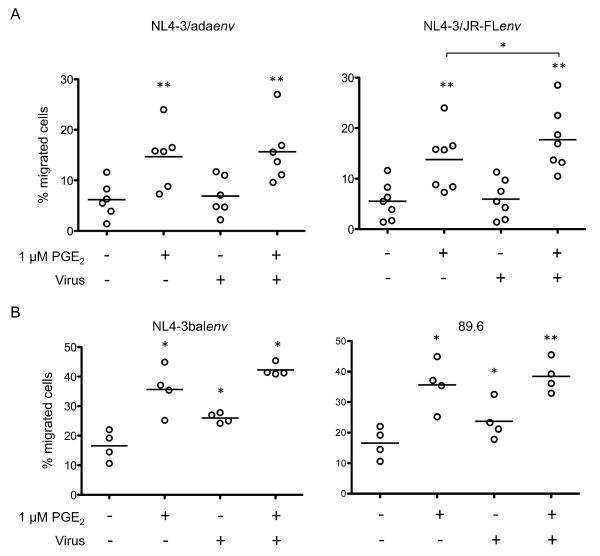
.
Figure 2: PGE2 enhances CCL19-specific migration of HIV-1 exposed Mono-Mac-1
Mono-Mac-1 cells were exposed to the indicated strains of pseudotyped (A) or replication competent (B) viruses. HIV-1-exposed cells were left untreated or treated with 1 μM PGE2 for 24 hours and migration to CCL19 was evaluated using an in vitro chemotaxis assay. Results were obtained from at least four independent experiments. **p < 0.01; *p < 0.05.
View Figure 2
Binding of chemokines to their receptors causes a characteristic increases in cytosolic calcium, which is one of the earliest biochemical events that occur in response to chemokines [34]. Thus, we hypothesized that treatment of cells with the potent intracellular Ca2+ chelator, BAPTA/AM [35], would block the migration-promoting actions of PGE2. In cells treated with PGE2, we observed 19.7 ± 6.9% cell migration but pretreatment with 25 μM BAPTA/AM reduced migration to 2.9 ± 1.6% (Figure 3) without affecting cell viability (data not shown). These observations establish a primary role for intracellular calcium signaling in the PGE2-induced migration of Mono-Mac-1 cells in response to CCL19.
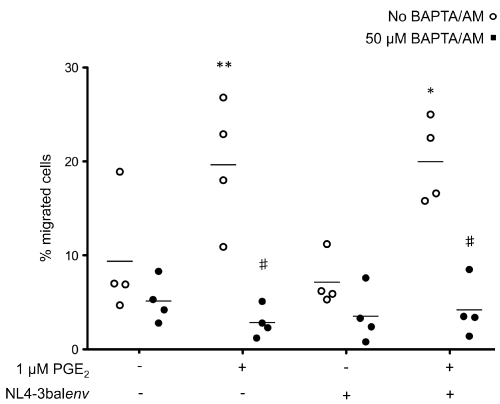
.
Figure 3: CCL19-specific migration of HIV-1-exposed Mono-Mac-1 is dependent of calcium
Mono-Mac-1 cells were exposed the indicated clone of HIV-1. HIV-1-exposed cells were left untreated or treated with 1 μM PGE2 for 24 hours. Cells were then treated with 50 μM BAPTA/AM or vehicle for 10 minutes and migration to CCL19 was evaluated using an in vitro chemotaxis assay. Each set of data represents a different blood donor. **p < 0.01; *p < 0.05 compared to mock-treated cells; #p < 0.01 compared to cells treated with BAPTA/AM.
View Figure 3
To further investigate the effects of PGE2 on CCR7-dependant migration of HIV-1-exposed monocytes, we repeated this series of experiments with freshly isolated human blood monocytes. These cells were exposed to different strains of pseudotyped viruses or replication competent HIV-1 clones and then HIV-1-exposed blood-isolated monocytes were left untreated or treated with PGE2 for 24 hours. Cells were then assessed for CCL19-specific migration as described above (Figure 4). While HIV-1 exposure reduced the number of migrating monocytes, PGE2-treatment significantly increased specific migration toward CCL19. Migration analysis of monocytes that were exposed to replication competent viruses showed significantly decreased numbers of migrating cells (Figure 4A compared with Figure 4B). However, the impaired migration observed in HIV-1-exposed cells was reversed by PGE2 treatment, resulting in migration levels corresponding to PGE2-treated uninfected monocytes. Importantly, our results indicate that HIV-1-exposition perturbed CCR7 expression in freshly isolated blood monocytes leading to a generalized defect of CCR7-dependent chemotaxis. This defect was reversed by the addition of PGE2. These data support the above results obtained by flow cytometry analysis of CCR7 surface expression observed in HIV-1-exposed blood monocytes treated with PGE2.
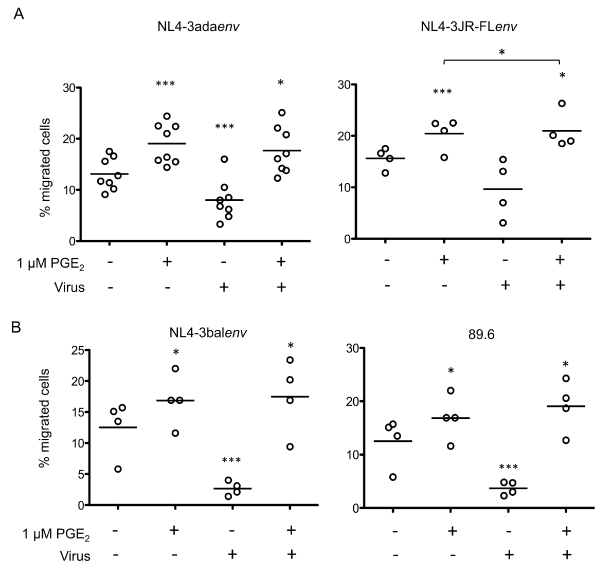
.
Figure 4: PGE2 enhances CCL19-specific migration of HIV-1-exposed monocytes
Freshly isolated blood monocytes were exposed to the indicated clones of pseudotyped (A) or replication competent (B) viruses. HIV-1-exposed cells were left untreated or treated with 1 μM PGE2 for 24 hours and migration to CCL19 was evaluated using an in vitro chemotaxis assay. Each set of data represents a different blood donor. ***p < 0.005; **p < 0.01; *p < 0.05.
View Figure 4
Next, we investigated whether the HIV-1 envelope glycoprotein directly regulated chemotaxis by exposing freshly isolated monocytes to the VSV-G pseudotyped virus NL4-3-Luc+E-R+/VSVenv. As shown in figure 5, exposition to virus particles where the gp120 was replaced by the VSV glycoprotein did not significantly reduce the percentage of migrated cells in the absence of PGE2 (uninfected cells: 12.8 ± 3.2% and NL4-3-Luc+E-R+/VSVenv infected cells: 10.9 ± 6.7%). Our results suggest that the interaction of gp120 with its receptor (s) on monocytes could be implicated in CCR7-driven migration.
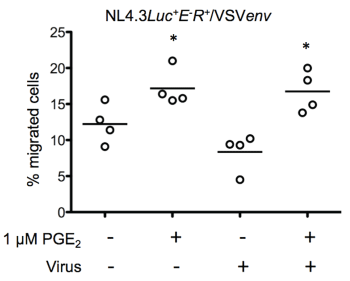
.
Figure 5: HIV-1 envelope proteins regulate CCR7-dependent chemotaxis
Freshly isolated blood monocytes were exposed to VSV-G pseudotyped HIV-1. Virus-exposed cells were left untreated or treated with 1 μM PGE2 for 24 hours and migration to CCL19 was evaluated using an in vitro chemotaxis assay. Each set of data represents a different blood donor. *p < 0.01.
View Figure 5
HIV-1-exposed monocytes can transfer infection to susceptible cells
Finally, we evaluated whether HIV-1-exposed monocytes that migrated in response to CCL19 were able to efficiently spread the virus to TZM-bl indicator cells (Figure 6). Treatment of NL4.3balenv-exposed monocytes with PGE2 significantly increased the relative infection indice (RLU/number of migrated cells in the lower chamber of the Transwell) compared to untreated HIV-1-exposed monocytes (Figure 6). Together the data demonstrated that PGE2 considerably regulates the capacity of HIV-1-exposed monocytes to migrate toward CCL19 and transmit the virus to uninfected host cells.
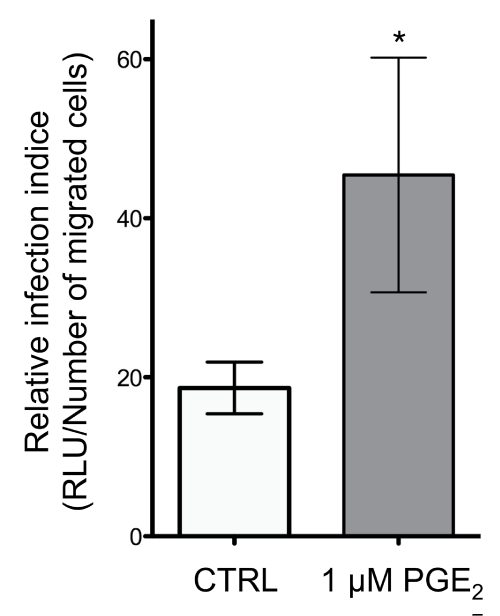
.
Figure 6: HIV-1-loaded monocytes responding to CCL19 can transfer HIV to susceptible cells in the presence of PGE2
Mono-Mac-1 cells were exposed to replication competent HIV NL4.3balenv and left untreated or treated with 1 μM PGE2 for 24 hours. Cells were subjected to the in vitro chemotactic assay using CCL19 and the migrated cells were added to the TZM-bl indicator cell line. Cells were co-cultured for 2 hours, the inoculum was washed off, and cells were cultured for an additional 48 hours. The luciferase activity in the TZM-bl cells was evaluated. *p < 0.05.
View Figure 6
Discussion
Monocytes are recognized as multifunctional contributors to immune function but many questions remain to be answered, such as how monocytes acquire trafficking and migration behaviors. Although it is controversial that monocytes are susceptible to HIV-1, several studies have reported that circulating monocytes are indeed infected in vivo and can serve as important reservoirs of HIV-1, especially in patients with late-stage disease and opportunistic infections [1,6-12,36]. Monocytes are regarded as early targets for HIV infection and as an important viral reservoir. Indeed, infected monocytes are important short-lived viral pool that upon entering tissues differentiated into macrophages (reviewed in [37]) On the other hand, HIV infection causes an early increase and a late decrease of CCL19 and CCL21 that are associated with disease progression [38-40], HIV replication in T cells [41,42], as well as the release of inflammatory mediators [39]. However, the impact of HIV-1 exposure on CCR7 expression in monocytes was still not characterized. Here, we have shown for the first time that exposition of freshly isolated human monocytes to R5 and dual-tropic HIV-1 altered CCR7-dependent migration in response to CCL19.
Firstly, this study demonstrated that HIV-1-exposition of human monocytes impaired CCR7-dependent migration in response to CCL19 and this required gp120 binding to human monocytes. This disruption of CCR7-dependent monocyte chemotactic function in HIV-1 infection may have major consequences on monocyte circulation given that CCR7 play an essential role in immune cells entry to lymph nodes. Due to defective CCR7 functions, monocytes exposed to HIV are likely to enter and circulate within peripheral tissues less efficiently, leading to decreased recognition pattern. The virus, to favour its dissemination, may exploit this mechanism. Furthermore and in concordance with our study, HIV infection was associated with reduced T-cell migration in response to CCL19 [43]. It was also shown that, in spite of preserved CCR7 expression, CCR7-dependent chemotactic responses were significantly decreased within most T-cell subsets from viremic patients, including naive, central memory, and effector memory CD4+ T cells and naive, central memory, and effector CD8+ T cells. Further, this defect in T-cell chemotaxis was only partially corrected by effective HAART treatment [43]. Our data are also supported by an investigation identifying a novel defect in CCR7 expression of plasmacytoid dendritic cells (pDCs) in pediatric patients with progressive HIV disease. The failure of CCR7 up-regulation was associated with failure of immune reconstitution and with ongoing plasma virus replication [44]. They proposed that failure to up-regulate CCR7 could be an impediment to the homing of pDCs to lymphoid tissue resulting in impaired maturation of myeloid DCs and impaired T-cell activation. In addition, it has been recently shown that respiratory syncytial virus infection can dowregulate CCR7 expression in newborns leading to delayed innate and adaptive immune activation [45]. Taken together, these data suggests that HIV has an important impact on cell circulation but the mechanism of HIV inhibition of CCR7 functionality remains to be fully defined.
Secondly, because serum levels PGE2 is a hallmark of the progression of HIV infection and contributes HIV pathogenesis, we also investigated its effect on the migratory capacity of HIV-exposed-monocytes. We assessed both the cell surface expression of CCR7 and CCR7 response to its ligand CCL19. As previously observed, we confirmed that PGE2 induced functional CCR7 expression on blood monocytes and Mono-Mac-1 cells, enabling them to respond to and migrate toward CCL19. Interestingly, PGE2-treatment of HIV-exposed blood-isolated monocytes restored their potential to migrate in response to CCL19 to a level comparable to PGE2-treated unexposed monocytes. The cellular and molecular mechanisms responsible for this are being pursued in our laboratory. Since CD16+ monocytes are sensitive to HIV-1 infection and they serve as a major virus reservoir [8,46], restoration of CCR7 function due to PGE2 may influence the pattern of cell recirculation, allowing cells to be directed to lymph nodes, Peyer's patches as well as other sites [9,13,15-18]. Moreover in our study, we also demonstrate that monocytes exposed to HIV-1 in the presence of PGE2 transfer HIV-1 particles to uninfected susceptible cells in response to CCL19. These findings may have important implications in HIV-1 dissemination to anatomical reservoirs. Indeed, interactions between prostaglandins, leukotrienes and HIV-1 may modulate HIV infection and contribute to the viral dissemination to anatomical reservoirs such as the central nervous system (reviewed in [47]).
The demonstration that CCR7 expression and functionality is restored by PGE2 in monocytes is not a universal phenomenon because chemokines receptors for other chemoattractants such as CCR5, CCR2 or CXCR4 are not affected by the same manner. The expression of CCR5, the receptor for RANTES, which may affect not only leukocyte migration, but also infectivity by HIV-1, has been shown to be down-regulated by PGE2 in monocytes [48,49] whereas CCR2 and CXCR4 expression were not affected [49]. While Thivierge et al. [48] showed that exposure to PGE2 reduced chemotaxis of monocytes in response to MIP-1β, Panzer and Uguccioni [49] clearly demonstrated that PGE2 enhances the migration in response to MCP-1, RANTES and SDF-1. Altogether, these data indicate that in inflammatory conditions, the up-regulated production of PGE2 by monocytes or tissue cells might favor the migratory capacity towards locally expressed inflammatory chemokines. By extension, monocytes in close contact with HIV-1 or infected by HIV-1 might disseminate the virus throughout the body.
Conclusion
In summary, our findings suggest a complex interplay between HIV and PGE2 for the expression of functional CCR7 on monocytes. In one hand, HIV exposition impairs CCR7-specific migration in monocytes. In the other hand, PGE2 found in serum of HIV-1 infected individuals in concentrations that increase over the time, restores the migratory capacity of HIV-1-exposed monocytes to CCL19. Our data reinforce the concept that PGE2 is a key mediator in CCR7 functionality. Since CCL19 and CCL21 production is induced in early phases of HIV-1 infection and serum level of PGE2 is amplified, circulating HIV-1-loaded monocytes may accumulated in peripheral lymph nodes as well as in other sanctuary sites such as brain where they can spread the infection to other susceptible cells.
Acknowledgement
We are very grateful to Dr. M.J. Tremblay (Université Laval) for his support. All the experiments using infectious clones were performed in his laboratory.
Authors' contributions
SCC performed the experiments, prepared the figures, analyzed the data and drafted the manuscript. SP carried out the transfections and viruses production. ND conceived the study, participated in its design and coordination and wrote the manuscript. All authors read and approved the final manuscript.
Conflict of interest
The authors declare they have no competing interests.
Funding
This study was supported by the Canadian Institutes of Health Research (CIHR) (Grant no. 126785).
References
-
Gartner S, Markovits P, Markovitz DM, Kaplan MH, Gallo RC, et al. (1986) The role of mononuclear phagocytes in HTLV-III/LAV infection. Science 233: 215-219.
-
Gendelman HE, Baca LM, Husayni H, Turpin JA, Skillman D, et al. (1990) Macrophage-HIV interaction: viral isolation and target cell tropism. AIDS 4: 221-228.
-
Coleman CM, Wu L (2009) HIV interactions with monocytes and dendritic cells: viral latency and reservoirs. Retrovirology 6: 51.
-
McElrath MJ, Steinman RM, Cohn ZA (1991) Latent HIV-1 infection in enriched populations of blood monocytes and T cells from seropositive patients. J Clin Invest 87: 27-30.
-
Sonza S, Mutimer HP, Oelrichs R, Jardine D, Harvey K, et al. (2001) Monocytes harbour replication-competent, non-latent HIV-1 in patients on highly active antiretroviral therapy. AIDS 15: 17-22.
-
Lambotte O, Taoufik Y, de Goër MG, Wallon C, Goujard C, et al. (2000) Detection of infectious HIV in circulating monocytes from patients on prolonged highly active antiretroviral therapy. J Acquir Immune Defic Syndr 23: 114-119.
-
Zhu T, Muthui D, Holte S, Nickle D, Feng F, et al. (2002) Evidence for human immunodeficiency virus type 1 replication in vivo in CD14(+) monocytes and its potential role as a source of virus in patients on highly active antiretroviral therapy. J Virol 76: 707-716.
-
Ellery PJ, Tippett E, Chiu YL, Paukovics G, Cameron PU, et al. (2007) The CD16+ monocyte subset is more permissive to infection and preferentially harbors HIV-1 in vivo. J Immunol 178: 6581-6589.
-
McElrath MJ, Pruett JE, Cohn ZA (1989) Mononuclear phagocytes of blood and bone marrow: comparative roles as viral reservoirs in human immunodeficiency virus type 1 infections. Proc Natl Acad Sci USA 86: 675-679.
-
Bahner I, Kearns K, Coutinho S, Leonard EH, Kohn DB (1997) Infection of human marrow stroma by human immunodeficiency virus-1 (HIV-1) is both required and sufficient for HIV-1-induced hematopoietic suppression in vitro: demonstration by gene modification of primary human stroma. Blood 90: 1787-1798.
-
Weiser B, Burger H, Campbell P, Donelan S, Mladenovic J (1996) HIV type 1 RNA expression in bone marrows of patients with a spectrum of disease. AIDS Res Hum Retroviruses 12: 1551-1558.
-
Rich EA, Chen IS, Zack JA, Leonard ML, O'Brien WA (1992) Increased susceptibility of differentiated mononuclear phagocytes to productive infection with human immunodeficiency virus-1 (HIV-1). J Clin Invest 89:176-183.
-
Wiley CA, Schrier RD, Nelson JA, Lampert PW, Oldstone MB (1986) Cellular localization of human immunodeficiency virus infection within the brains of acquired immune deficiency syndrome patients. Proc Natl Acad Sci USA 83:7089-7093.
-
Orenstein JM, Fox C, Wahl SM (1997) Macrophages as a source of HIV during opportunistic infections. Science 276: 1857-1861.
-
Ivey NS, MacLean AG, Lackner AA (2009) Acquired immunodeficiency syndrome and the blood-brain barrier. J Neurovirol 15: 111-122.
-
Chayt KJ, Harper ME, Marselle LM, Lewin EB, Rose RM, et al. (1986) Detection of HTLV-III RNA in lungs of patients with AIDS and pulmonary involvement. JAMA 256: 2356-2359.
-
Armstrong JA, Horne R (1984) Follicular dendritic cells and virus-like particles in AIDS-related lymphadenopathy. Lancet 2: 370-372.
-
Atta MG, Lucas GM, Fine DM (2008) HIV-associated nephropathy: epidemiology, pathogenesis, diagnosis and management. Expert Rev Anti Infect Ther 6: 365-371.
-
Saeki H, Moore AM, Brown MJ, Hwang ST (1999) Cutting edge: secondary lymphoid-tissue chemokine (SLC) and CC chemokine receptor 7 (CCR7) participate in the emigration pathway of mature dendritic cells from the skin to regional lymph nodes. J Immunol 162: 2472-2475.
-
Stein JV, Rot A, Luo Y, Narasimhaswamy M, Nakano H, et al. (2000) The CC chemokine thymus-derived chemotactic agent 4 (TCA-4, secondary lymphoid tissue chemokine, 6Ckine, exodus-2) triggers lymphocyte function-associated antigen 1-mediated arrest of rolling T lymphocytes in peripheral lymph node high endothelial venules. J Exp Med 191: 61-76.
-
Warnock RA, Campbell JJ, Dorf ME, Matsuzawa A, McEvoy LM, et al. (2000) The role of chemokines in the microenvironmental control of T versus B cell arrest in Peyer's patch high endothelial venules. J Exp Med 191: 77-88.
-
Campbell JJ, Murphy KE, Kunkel EJ, Brightling CE, Soler D, et al. (2001) CCR7 expression and memory T cell diversity in humans. J Immunol 166: 877-884.
-
Côté SC, Pasvanis S, Bounou S, Dumais N (2009) CCR7-specific migration to CCL19 and CCL21 is induced by PGE(2) stimulation in human monocytes: Involvement of EP(2)/EP(4) receptors activation. Mol Immunol 46: 2682-2693.
-
Förster R, Schubel A, Breitfeld D, Kremmer E, Renner-Müller I, et al. (1999) CCR7 coordinates the primary immune response by establishing functional microenvironments in secondary lymphoid organs. Cell 99: 23-33.
-
Gunn MD, Kyuwa S, Tam C, Kakiuchi T, Matsuzawa A, et al. (1999) Mice lacking expression of secondary lymphoid organ chemokine have defects in lymphocyte homing and dendritic cell localization. J Exp Med 189: 451-460.
-
Scandella E, Men Y, Legler DF, Gillessen S, Prikler L, et al. (2004) CCL19/CCL21-triggered signal transduction and migration of dendritic cells requires prostaglandin E2. Blood 103: 1595-1601.
-
Scandella E, Men Y, Gillessen S, Förster R, Groettrup M (2002) Prostaglandin E2 is a key factor for CCR7 surface expression and migration of monocyte-derived dendritic cells. Blood 100: 1354-1361.
-
Abel PM, McSharry C, Galloway E, Ross C, Severn A, et al. (1992) Heterogeneity of peripheral blood monocyte populations in human immunodeficiency virus-1 seropositive patients. FEMS Microbiol Immunol 5: 317-323.
-
Foley P, Kazazi F, Biti R, Sorrell TC, Cunningham AL (1992) HIV infection of monocytes inhibits the T-lymphocyte proliferative response to recall antigens, via production of eicosanoids. Immunology 75: 391-397.
-
Griffin DE, Wesselingh SL, McArthur JC (1994) Elevated central nervous system prostaglandins in human immunodeficiency virus-associated dementia. Ann Neurol 35: 592-597.
-
Platt EJ, Wehrly K, Kuhmann SE, Chesebro B, Kabat D (1998) Effects of CCR5 and CD4 cell surface concentrations on infections by macrophagetropic isolates of human immunodeficiency virus type 1. J Virol 72: 2855-2864.
-
Bounou S, Leclerc JE, Tremblay MJ (2002) Presence of host ICAM-1 in laboratory and clinical strains of human immunodeficiency virus type 1 increases virus infectivity and CD4(+)-T-cell depletion in human lymphoid tissue, a major site of replication in vivo. J Virol 76:1004-1014.
-
Boisvert M, Côté S, Vargas A, Pasvanis S, Bounou S, et al. (2008) PGJ2 antagonizes NF-kappaB-induced HIV-1 LTR activation in colonic epithelial cells. Virology 380: 1-11.
-
Müller A, Homey B, Soto H, Ge N, Catron D, et al. (2001) Involvement of chemokine receptors in breast cancer metastasis. Nature 410: 50-56.
-
Harrison SM, Bers DM (1987) The effect of temperature and ionic strength on the apparent Ca-affinity of EGTA and the analogous Ca-chelators BAPTA and dibromo-BAPTA. Biochim Biophys Acta 925: 133-143.
-
Cassol E, Alfano M, Biswas P, Poli G (2006) Monocyte-derived macrophages and myeloid cell lines as targets of HIV-1 replication and persistence. J Leukoc Biol 80: 1018-1030.
-
Kumar A, Abbas W, Herbein G (2014) HIV-1 latency in monocytes/macrophages. Viruses 6: 1837-1860.
-
Damas JK, Oktedalen O, Ueland T, Landro L, Barstad J, et al. (2012) Enhanced levels of CCL19 in patients with advanced acquired immune deficiency syndrome (AIDS). Clin Exp Immunol 167: 492-498.
-
Damas JK, Landro L, Fevang B, Heggelund L, Tjonnfjord GE, et al. (2009) Homeostatic chemokines CCL19 and CCL21 promote inflammation in human immunodeficiency virus-infected patients with ongoing viral replication. Clin Exp Immunol 157: 400-407.
-
Fontaine J, Poudrier J, Roger M (2011) Short communication: persistence of high blood levels of the chemokines CCL2, CCL19, and CCL20 during the course of HIV infection. AIDS Res Hum Retroviruses 27: 655-657.
-
Nagira M, Sato A, Miki S, Imai T, Yoshie O (1999) Enhanced HIV-1 replication by chemokines constitutively expressed in secondary lymphoid tissues. Virology 264: 422-426.
-
Saleh S, Solomon A, Wightman F, Xhilaga M, Cameron PU, et al. (2007) CCR7 ligands CCL19 and CCL21 increase permissiveness of resting memory CD4+ T cells to HIV-1 infection: a novel model of HIV-1 latency. Blood 110: 4161-4164.
-
Perez-Patrigeon S, Vingert B, Lambotte O, Viard JP, Delfraissy JF, et al. (2009) HIV infection impairs CCR7-dependent T-cell chemotaxis independent of CCR7 expression. AIDS 23: 1197-1207.
-
Desai S, Chaparro A, Liu H, Haslett P, Arheart K, et al. (2007) Impaired CCR7 expression on plasmacytoid dendritic cells of HIV-infected children and adolescents with immunologic and virologic failure. J Acquir Immune Defic Syndr 45: 501-507.
-
Inchley CS, Osterholt HC, Sonerud T, Fjærli HO, Nakstad B (2013) Downregulation of IL7R, CCR7, and TLR4 in the cord blood of children with respiratory syncytial virus disease. J Infect Dis 208: 1431-1435.
-
Ancuta P, Kunstman KJ, Autissier P, Zaman T, Stone D, et al. (2006) CD16+ monocytes exposed to HIV promote highly efficient viral replication upon differentiation into macrophages and interaction with T cells. Virology 344: 267-276.
-
Bertin J, Barat C, Méthot S, Tremblay, MJ (2012) Interactions between prostaglandins, leukotrienes and HIV-1: Possible implications for the central nervous system. Retrovirology 9: 4-18.
-
Thivierge M, Le Gouill C, Tremblay MJ, Stankova J, Rola-Pleszczynski M (1998) Prostaglandin E2 induces resistance to human immunodeficiency virus-1 infection in monocyte-derived macrophages: downregulation of CCR5 expression by cyclic adenosine monophosphate. Blood 92: 40-45.
-
Panzer U, Schneider A, Wilken J, Thompson DA, Kent SB, et al. (1999) The chemokine receptor antagonist AOP-RANTES reduces monocyte infiltration in experimental glomerulonephritis. Kidney Int 56: 2107-2115.
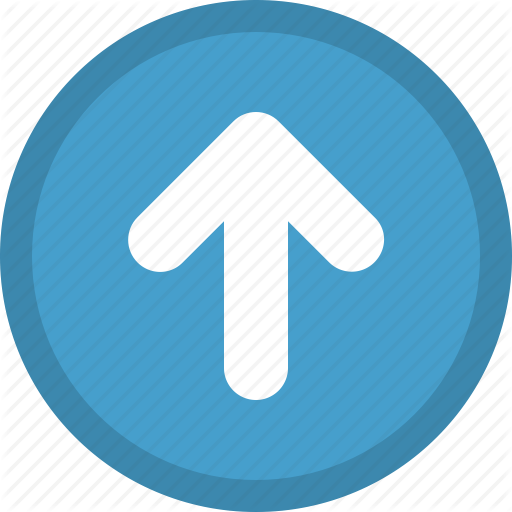