Journal of Otolaryngology and Rhinology
Comparison of Color Flow Doppler, Angiogram, CT Angiogram and Time Resolved MR Angiogram in the Preoperative Evaluation of Lower Extremity Vascularity for Fibular Free Flap Reconstruction
Catherine Weng, Rod Rezaee*, Jason E Thuener and Chad Zender
Department of Otolaryngology, Head and Neck Surgery, Case Western Reserve University & University Hospitals Case Medical Center, Cleveland, OH, USA
*Corresponding author:
Rod Rezaee, MD, Department of Otolaryngology, Head and Neck Surgery, Case Western Reserve University & University Hospitals Case Medical Center, Cleveland, OH, USA, E-mail: Rod.Rezaee@uhhospitals.org
J Otolaryngol Rhinol, JOR-2-011, (Volume 2, Issue 1), Retrospective Chart Review
Received: August 10, 2015: Accepted: January 25, 2016: Published: January 27, 2016
Citation: Weng C, Rezaee R, Thuener JE, Zender C (2016) Comparison of Color Flow Doppler, Angiogram, CT Angiogram and Time Resolved MR Angiogram in the Preoperative Evaluation of Lower Extremity Vascularity for Fibular Free Flap Reconstruction. J Otolaryngol Rhinol 2:011
Copyright: © 2016 Weng C, et al. This is an open-access article distributed under the terms of the Creative Commons Attribution License, which permits unrestricted use, distribution, and reproduction in any medium, provided the original author and source are credited.
Abstract
Importance:Various imaging modalities have been used to evaluate lower extremity anatomy including traditional angiography, color flow Doppler (CFD), CT angiography (CTA) and MR angiography (MRA).
Objective:We seek to compare these imaging modalities for preoperative planning of fibular osteocutaneous free flap reconstruction and to identify the optimal imaging method.
Design, Setting and Participants:Between 2002 and 2013 the senior authors performed over 600 adult microvascular free tissue transfers. 161 of those patients had a fibular osteocutaneous free flap requiring lower extremity imaging. Retrospective chart review was performed on the imaging findings, surgical procedures and postoperative course of these patients. One patient was excluded since we did not have complete information regarding preoperative imaging.
Intervention:Preoperative imaging was performed as per standard practices of the two senior authors.
Main outcome Measures:Imaging findings, operative reports, postoperative outcomes and complications were reviewed from the medical records according to an Institutional Review Board approved protocol. Statistical analysis was performed using 2 tailed students T test using the Prism 6 software.
Results:160 patient charts were reviewed. Patients' ages ranged from 20-92, with 105 males, and 55 females. Preoperative evaluation included 18 angiograms, 19 CTAs, 21 CFDs and 102 with MRAs. Relevant anatomic abnormalities, including atherosclerosis or aberrant anatomy, were identified in the anterior tibial artery (ATA), posterior tibial artery (PTA) or peroneal artery in 10 (55%) patients receiving angiogram, 10 (52%) receiving CTA, 3 (14%) receiving CFD, and 50 (49%) receiving MRA. There was a statistically significant difference in the identification of abnormality between the CFD group and the angiogram (P = 0.015), CTA (P = 0.017), and MRA (P = 0.041) groups. However, there was no statistical difference among the other three groups.
Conclusions and Relevance:We conclude that CFD is less likely to detect anatomic abnormalities in the lower extremities when compared with angiogram, CTA or MRA. This suggests that CFD may not provide sufficient data for preoperative planning of fibular free flap harvest. However, because donor site ischemia is so rare, a larger prospective study may be necessary to identify a useful difference in relevant findings affecting complication rates.
Background
Since its initial description in the 1970's [1], and after gaining popularity in the late 1980's and early 90's by Hidalgo, the fibular free flap has become the most widely used method for reconstructing osteocutaneous defects of the head and neck. The fibula is a favored donor site because of the low rate of donor site morbidity, strong bone stock, reliable blood supply and flexible geometry of the skin paddle [2]. In order to safely and successfully perform fibular free flap transfer, the lower extremity blood supply must be carefully evaluated to ensure optimal anatomic requirements.
The most catastrophic but preventable donor site complication with fibular free flap transfer is distal foot ischemia [3,4]. Applying proper knowledge of lower extremity arteriovascular anatomy and avoiding fibular free flap harvest where it would compromise perfusion to the foot can prevent this complication. In the most common configuration, which is present in 88% of the population, the popliteal artery bifurcates into the tibioperoneal trunk and the anterior tibial artery (ATA) [3]. Then, the tibioperoneal trunk further bifurcates into the posterior tibial artery (PTA) and the peroneal artery. The peroneal artery typically stops at the ankle, and along its course sends off septocutaneous perforators to the skin of the lower leg. The dorsum of the foot, including the dorsalis pedis artery, is supplied by the ATA while the lateral plantar artery and deep plantar arch are supplied by the PTA [3]. In order to safely perform fibular harvest and avoid foot ischemia, preoperative imaging should confirm that the foot is supplied by 2 arteries other than the peroneal [3]. Congenital anatomic variants exist that would preclude the use of a particular leg for fibula free flap harvest. For example, the peroneal artery is sometimes the dominant supply to the foot, a condition called peroneal artery magna [3]. In other cases, the peroneal artery can be absent. Anomalous branching patterns at the trifurcation of the popliteal to ATA, PTA and peroneal arteries can occur in 7-12% of the population [5]. Peroneal artery magna can occur in 0.2-5.3% of the population [5].
Additionally, because of the high incidence of smoking and alcohol consumption in head and neck cancer patients, these patients are also at increased risk for atherosclerotic disease which can compromise lower extremity perfusion. The presence of peripheral arterial disease in the ATA and PTA can result in formation of collaterals resulting in a dominant peroneal artery perfusion to the foot. The ideal candidate for fibular free flap transfer is free of moderate or severe stenosis in each of the three vessels with a non-dominant peroneal artery and the presence of at least one septocutaneous perforator [6].
While controversy exists over the best method for preoperative lower extremity assessment, most surgeons agree that some form of imaging is necessary [7]. Each method has its strengths and limitations and there have been proponents for each in the literature. Angiography utilizes X-ray in multiple planes after administration of contrast and then through digital subtraction is able to produce an image of the lower extremity vasculature with excellent resolution [8,9]. For many years this was considered the gold standard of the imaging techniques [9,10]. Angiography provides adequate information about lower extremity arterial configuration and atherosclerotic disease. However, angiography is invasive in nature and carries a 3-5% risk of complications including arterial occlusion, hematoma, aortic dissection, intimal damage, pseudoaneurysm at the groin site, contrast allergy, and nephrotoxicity [8,11]. Additional disadvantages of angiography include exposure to radiation, contrast dye exposure, and postprocedural care that may require overnight hospitalization [8]. Color flow Doppler (CFD) is a noninvasive and inexpensive method that uses ultrasound principles to record blood flow and velocity in the extremity [8]. CFD is operator dependent, time intensive, and may not provide enough anatomic detail. CT angiography (CTA) is performed after injection of a bolus of iodinated contrast dye [8]. CTA is non-invasive and it provides an accurate depiction of the course and caliber of vessels in 2 and 3 dimensional reconstructions for surgical planning with enough detail to follow the course of the perforators [8]. However, radiation exposure associated with CTA is estimated at 5.6 mSv and exposure to contrast dye can result in allergic reaction, nephrotoxicity and local vessel spasm [8]. Artifact from calcification of the vessel wall can obscure the image and affect interpretation in CTA [12]. Magnetic resonance angiography (MRA) uses a high power magnetic field and radio frequency pulses to align hydrogen nuclei, which is then detected by a scanner [8]. MRA, particularly with time resolved contrast protocol, has the advantages of avoiding radiation exposure and limited contrast use. However, MRA is time consuming, expensive, and unattainable in some patients with incompatible implants. Figure 1 shows examples of both normal and abnormal MRA and CTA studies of the lower extremities. In both of the abnormal studies, the surgical plan would be influenced by the findings demonstrated in preoperative imaging.
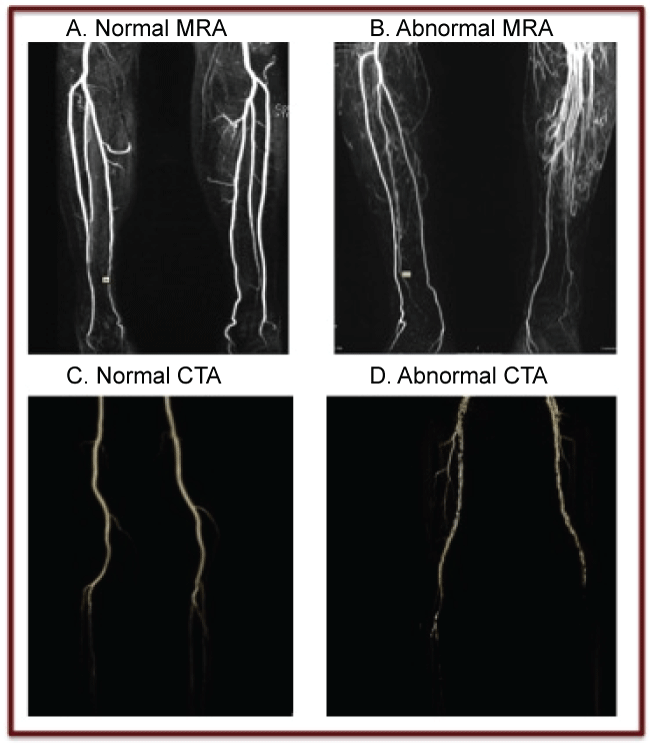
.
Figure 1: A. Normal MRA B. Abnormal MRA: Left ATA patent only to mid-calf and peroneal supplies foot with collaterals, Right normal C. Normal CTA D. Abnormal CTA Left peroneal dominant supply to foot, Right atherosclerosis in 3 vessels.
View Figure 1
Methods
Between 2002 and 2013 the senior authors performed over 600 adult microvascular free tissue transfers. 161 of those patients were planned to have a fibula osteocutaneous free flap requiring lower extremity imaging. One patient was excluded due to incomplete information regarding preoperative imaging. Preoperatively each of these patients received lower extremity imaging as per standard practices of the two senior authors. During the study time, the predominant imaging modality at our institution changed from angiography and CFD to time resolved MRA and CTA. All patients were imaged with the goal of identifying anatomic configurations that would contraindicate fibular harvest due to congenital variation or arteriovascular disease.
An Institutional Review Board approved protocol was used for retrospective chart review of the electronic and paper medical record of eligible patients. Imaging findings, operative reports, postoperative course and complications were all reviewed and all data was deidentified.
Data used for comparison of the various imaging modalities included the presence of an abnormality relevant to fibular harvest, laterality of the flap harvest site, postoperative complications and whether these complications could have resulted from the imaging abnormality identified. Relevant imaging abnormalities included significant atherosclerosis in the peroneal, ATA or PTA and any indication of aberrant supply of the foot including peroneal artery magna. Significant disease was defined as loss of patency to the foot of the affected vessel. The laterality of the flap harvest site was recorded and if it was chosen on the side with more favorable vascular imaging, it was concluded that the imaging helped to determine surgical plan. Statistical analysis was performed using 2-sided Fisher exact test using the Prism 6 software.
Results
Demographics
160 patient charts were reviewed. Patients' ages ranged from 20-92, with 105 males and 55 females. The mean and median age was 61. Preoperative evaluation included 18 angiograms, 19 CTAs, 21 CFDs, and 102 with MRAs (Table 1).
Table 1: Demographics.
View Table 1
Imaging abnormalities
Relevant anatomic abnormalities, including atherosclerosis or aberrant anatomy, were identified in the anterior tibial artery (ATA), posterior tibial artery (PTA) or peroneal artery in 10 (55%) patients receiving angiogram, 10 (52%) receiving CTA, 3 (14%) receiving CFD, and 50 (49%) receiving MRA (Table 2). There was a statistically significant difference in identification of abnormality between the CFD group and the angiogram (P = 0.015), CFD and CTA (P = 0.017), and CFD and MRA (P = 0.041) groups (Table 3). However, there was no statistical difference among the other three groups.
Table 2: Comparison of Angiogram CTA, CFD and MRA.
View Table 2
Table 3: Statistical significance of abnormality detection of imaging methods.
View Table 3
Effect on surgical decision-making
Abnormalities identified in the preoperative imaging could lead to choosing a contralateral leg, or aborting fibular free flap reconstruction all together and proceeding with a different free flap or local pedicled flap reconstruction. The abnormal arteriovascular anatomy identified in the preoperative imaging affected laterality of flap harvest in 5 (27%) patients receiving angiogram, 8 (42%) patients receiving CTA, 3 (14%) patients receiving CFD, and 31 (30%) patients receiving MRA (Table 2). There was no statistically significant difference between groups. CFD does appear to have influenced the surgical plan less often, but this was not statistically significant (Table 3).
Complications
There were only two postoperative donor site complications, and both were related to wound infections that were managed conservatively. There were no cases of vascular insufficiency to the donor site extremity. Flap related complications at the recipient site included hematoma, anastamotic thrombosis, and flap failure, fistula and wound infection. There were 3 (17%) in the angiogram group, 5 (26%) in the CTA group, 8 (38%) in the CFD group, and 28 (27%) in the MRA group (Table 2). Within the angiogram group, complications included one hematoma at postoperative day 10, and two cases with distal skin flap congestion requiring leech therapy. Among the CTA group, there were three cases of flap dehiscence or fistula formation, one case of skin necrosis requiring leech therapy and one hematoma at postoperative day one requiring evacuation. Among the CFD group, there were 6 cases of wound dehiscence and fistula formation, one surgical site infection involving the flap and one case of wound break down leading to facial artery rupture requiring urgent surgical exploration. There was no statistical difference in complication rates between groups based on imaging modality. In the MRA group there were 7 hematomas, 8 wound infections (one of which led to delayed flap failure), 8 wound dehiscence/fistula, two early flap failures requiring reoperation, one early seroma leading to flap necrosis requiring washout and debridement, and one flap that was discovered to be non-salvageable intraoperatively requiring use of a different reconstructive method. Given that these complications were at the recipient site and were likely multifactorial, it would be difficult to assign causation related to imaging abnormalities. Other general medical complications including postoperative pulmonary embolism, myocardial infarction, pneumonia and other infections not related to the surgical site were not analyzed.
Discussion
While numerous studies have compared one preoperative imaging method to another [2,10,11,13-16] we believe our study is the first to compare all 4 imaging modalities head to head in assessing their impact on fibular free flap planning and outcome.
Given the relatively small sample size, we elected to perform 2 tailed T tests comparing each pair of groups with different imaging. Also, given the assumption that the ability of each imaging modality to identify abnormal arterial supply should be independent of any other modality, we elected to compare each pair separately with individual T tests. We have demonstrated that CFD is less likely to identify a significant anatomic abnormality than the other three imaging modalities. Our data also seems to imply that CFD results are less likely to influence the operative plan than the other modalities studied. We hypothesize this is because fewer abnormalities are identified by CFD. We believe that significantly fewer abnormalities are found on CFD because it is not sensitive enough to identify less severe abnormalities. Alternatively, the other modalities are too sensitive resulting in identification of subclinical abnormalities. This is also reflected in the data since CFD results influenced surgical plan in 14% of cases compared to 27-42% in the other groups. However, this difference was not statistically significant and the different modalities should be analyzed in a prospective study with a larger sample size.
Overall, we found CFD inferior in quality and accuracy when compared to the other imaging modalities. As previously described, disadvantages of CFD include its inability to provide a comprehensive map of the lower extremity blood supply for surgical planning, inability to provide internal vessel diameter and variability in results depending on the experience of the technician performing the study [8]. Futran et al. examined 6 patients with known peripheral arterial disease (PAD) with both conventional angiography and CFD and found similar results. At the same time, they also performed preoperative CFD on 10 patients undergoing fibular free flap reconstruction and found no abnormalities in CFD. Postoperatively, there were no donor site complications and they concluded that CFD is an acceptable and cost effective mode of preoperative imaging for fibular free flap harvest [10,13]. However, Lorenz et al. were skeptical of this recommendation, pointing out that there have been no reports of CFD identifying pathology in patients prior to free flap surgery implying a possible false negative rate not identified in the published literature [2].
Ahmad et al. proposed the use of CFD to evaluate lower extremity vasculature only in the case of abnormal physical exam, such as non-palpable dorsalis pedis or posterior tibial pulse at the ankle [11]. They evaluated 16 patients prior to fibular transfer with physical exam only and reported no donor site complications thus concluding this was a safe method [11]. Further supporting the use of CFD, Smith et al. performed preoperative imaging with both CFD and angiography (believed to be gold standard) on 17 patients (34 legs) and found 18 legs with abnormal perfusion that would make them high risk for fibula harvest [9]. These abnormal legs demonstrated biphasic, monophasic or no flow waveforms and were confirmed to be abnormal on angiography [9]. The remaining 16 legs demonstrated normal anatomy with both modalities. The similarity of results between CFD and angiography in this study might reflect the operator dependence of CFD.
We found comparable results between the newer MRA methods, with traditional angiography and CTA. However, the disadvantages of MRA including higher cost, lower resolution compared to CTA (to 1 mm compared to 0.3 mm), incompatibility with certain implants (defibrillators, cochlear implants) and difficulty with patients who experience claustrophobia limit its application [8,12]. MRA resolution has improved with newer technology 1.5T (rather than 3T) and more recently blood pooled contrast material. Lorenz et al. evaluated 32 patients between 1996 and 2000 with preoperative MRA and identified 4 patients where arteriovascular disease or aberrant anatomy influenced the laterality of flap harvest [2]. In addition, they found 3 patients who were not suitable candidates for fibular harvest on both side and therefore required use of a different donor site. Overall, MRA results influenced the surgical planning in 21% of patients, with no reports of postoperative donor site morbidity supporting validity in fibula flap surgical planning [2]. Fukaya et al. also demonstrated the feasibility of mapping out peroneal anatomy, perforator location, and preoperative fibula flap planning with MRA in a series of studies [14,15]. No significant anatomic variants or significant peripheral vascular disease was identified in either study; however, 13 perforators were identified on MRA imaging with confirmation of 12 at the time of surgery. Lohan et al. reviewed 29 patients who received preoperative MRA for fibular free flap planning with 81% of subjects demonstrating normal three vessel runoff. Free flap donor site laterality was influenced by MRA in 13 patients, while 3 patients were not considered fibula flap candidates based on MRA results [7]. The authors also highlighted the cost efficiency of MRA when compared to angiography since postprocedural care and admission was unnecessary [7].
While reliable at detecting larger vessel disease or aberration, earlier MRA methods were not reliable at predicting perforator location and origin. A retrospective study of 123 patients that received MRA between 2004-2009 showed a correlation between perforators identified on imaging with surgically identified perforators only 10.9% of the time [4]. However, with ever evolving imaging technology, our ability to detect perforators has improved. A study recently published by one of our senior authors demonstrated high resolution mapping of perforators with time resolved MRA techniques in 27 patients [6].
A number of studies in the vascular medicine and surgery literature have systematically scrutinized comparison between CFD, CTA, MRA and angiography in the evaluation of carotid artery stenosis and peripheral arterial disease. Anzidei et al. evaluated 170 patients with history of stroke using CTA, MRA and color flow Doppler [17]. They found accuracy, sensitivity, and specificity of 97%, 95%, and 97% with CTA, 95%, 93% and 97% with MRA, and 76%, 67%, and 87% with CFD for carotid artery stenosis in a multicenter trial [17]. A Dutch group in 2008 assessed symptomatic patients with peripheral arterial disease (PAD) of the lower extremities using MRA, CTA and CFD. 514 patients with PAD were randomized to receive one of the 3 non-invasive methods for evaluation of their lower extremities [12]. They showed that CFD is not as sensitive as CTA or MRA, and although the initial cost is less, the final cost is actually more because of the necessary follow up imaging acquired due to insufficient data from CFD. In 2008, CFD cost 104 Euro, MRA cost 472 Euro, and CTA cost 163 Euro to perform [12].
The vascular surgery literature seems to be in agreement with our conclusion that CFD is less likely to find a significant arteriovascular abnormality than traditional angiography, MRA, or CTA. However, given the low rate of donor site complications in our study group and previous studies, the clinical implication of subtle vessel abnormalities is not fully understood. Future studies can be pursued to further delineate these differences.
In today's healthcare setting, cost can be a major factor when obtaining preoperative imaging. In 1997, Futran et al. compared the cost of angiography, MRA and CFD demonstrating CFD was less than half the cost of the other two methods: $295-300 for color flow Doppler compared to $696-758 for MRA and $786-892 for angiography [10]. Lorenz et al. found similar cost proportions in their study from 1996-2000. At that time the relative cost for angiography, MRA and CFD was 1:0.9: 0.4 respectively [2]. During our study period the Medicare fee for CFD was quoted as $176.33. In comparison, the fee for MRA was $537.58, CTA was $420.57 and angiography was $245.30. Microvascular surgeons must consider the benefit of accurate preoperative imaging for surgical planning when interpreting the cost of CTA and MRA. Although the price for each test has likely increased in the last eighteen years, new techniques have only improved each imaging modality.
We also recognize that the sizes of our groups are unequal. While this can be seen as a possible cause of selection bias for the imaging modality, it actually reflects some of the limitations of the retrospective nature of our study. Our sample of 160 patients includes patients from two different institutions over an 11-year span. One of the senior authors (RPR) initially worked at an institution where angiography and CTA were the preferred methods. In more recent years, our institution's radiology department favored use of MRA for the reasons described above. This was also the period where we had a significant increase in volume of fibular free flap surgeries with the addition of our second reconstructive surgeon (CAZ) resulting in a much higher patient number in the MRA group.
Conclusion
We conclude that CFD is less likely to detect anatomic abnormality in the lower extremities than angiogram, CTA or MRA. This suggests that CFD may not provide sufficient data for fibular free flap harvest. Trends from our data seem to indicate that CFD was less likely to influence the surgical plan. However, we do not have enough data to prove this with statistical significance. Also, we did not identify donor site ischemia among our patient cohort in any group. A larger prospective study may be necessary to identify clinically significant imaging findings that can predict complications.
Disclosure
The authors of this article have no disclosures, financial interest, or any other conflicts of interests to disclose.
References
- Taylor G (1983) The current status of free vascularized bone grafts. Clin Plast Surg 10: 185-209.
- Lorenz RR, Esclamado R (2001) Preoperative magnetic resonance angiography in fibular-free flap reconstruction of head and neck defects. Head Neck 23: 844-850.
- Kelly AM, Cronin P, Hussain HK, Londy FJ, Chepeha DB, et al. (2007) Preoperative MR angiography in free fibula flap transfer for head and neck cancer: clinical application and influence on surgical decision making. AJR Am J Roentgenol 188: 268-274.
- Miller ME, Moriarty JM, Blackwell KE, Finn JP, Yiee JH, et al. (2011) Preoperative magnetic resonance angiography detection of septocutaneous perforators in fibula free flap transfer. Arch Facial Plast Surg 13: 36-40.
- Betz LH, Betz BW (2009) Peronea arteria magna. Pediatr Radiol 39: 1016.
- Sandhu GS, Rezaee RP, Jesberger J, Wright K, Griswold MA, et al. (2012) Time-resolved MR angiography of the legs at 3 T using a low dose of gadolinium: initial experience and contrast dynamics. AJR Am J Roentgenol 198: 686-691.
- Lohan DG, Tomasian A, Krishnam M, Jonnala P, Blackwell KE, et al. (2008) MR angiography of lower extremities at 3 T: presurgical planning of fibular free flap transfer for facial reconstruction. AJR Am J Roentgenol 190: 770-776.
- Smit JM, Klein S, Werker PM (2010) An overview of methods for vascular mapping in the planning of free flaps. J Plast Reconstr Aesthet Surg 63: e674-682.
- Smith RB, Thomas RD, Funk GF (2003) Fibula free flaps: the role of angiography in patients with abnormal results on preoperative color flow Doppler studies. Arch Otolaryngol Head Neck Surg 129: 712-715.
- Futran ND, Stack BC Jr, Zaccardi MJ (1998) Preoperative color flow Doppler imaging for fibula free tissue transfers. Ann Vasc Surg 12: 445-450.
- Ahmad N, Kordestani R, Panchal J, Lyles J (2007) The role of donor site angiography before mandibular reconstruction utilizing free flap. J Reconstr Microsurg 23: 199-204.
- Ouwendijk R, de Vries M, Stijnen T, Pattynama PM, van Sambeek MR, et al. (2008) Multicenter randomized controlled trial of the costs and effects of noninvasive diagnostic imaging in patients with peripheral arterial disease: the DIPAD trial. AJR Am J Roentgenol 190: 1349-1357.
-
Futran ND, Stack BC Jr, Payne LP (1997) Use of color Doppler flow imaging for preoperative assessment in fibular osteoseptocutaneous free tissue transfer. Otolaryngol Head Neck Surg 117: 660-663.
- Fukaya E, Grossman RF, Saloner D, Leon P, Nozaki M, et al. (2007) Magnetic resonance angiography for free fibula flap transfer. J Reconstr Microsurg 23: 205-211.
- Fukaya E, Saloner D, Leon P, Wintermark M, Grossman RF, et al. (2010) Magnetic resonance angiography to evaluate septocutaneous perforators in free fibula flap transfer. J Plast Reconstr Aesthet Surg 63: 1099-1104.
- Futran ND, Stack BC Jr, Zachariah AP (1999) Ankle-arm index as a screening examination for fibula free tissue transfer. Ann Otol Rhinol Laryngol 108: 777-780.
- Anzidei M, Napoli A, Zaccagna F, Di Paolo P, Saba L, et al. (2012) Diagnostic accuracy of colour doppler ultrasonography, CT angiography and blood-pool-enhanced MR angiography in assessing carotid stenosis: A comparative study with DSA in 170 patients. Radiol Med 117: 54-71.
