International Journal of Cancer and Clinical Research
Isn't it the Timing for Physics Guidelines on High Dose Rate (HDR) Brachytherapy for Localized Prostate Cancer?
Yongbok Kim*
Department of Radiation Oncology, The University of Arizona, USA
*Corresponding author:
Yongbok Kim,Ph.D. Department of Radiation Oncology, The University of Arizona, 1501 N.Campbell Avenue, Tucson, AZ 85724, USA, PhoneTel: 520-694-7427, Fax:520-694-3215, Electronic-mail: yongbokkim@email.arizona.edu
Int J Cancer Clin Res, IJCCR-1-002, (Volume 1, Issue 1), Editorial; ISSN: 2378-3419
Received: August 15, 2014 | Accepted: August 21, 2014 | Published: August 23, 2014
Citation: Kim Y (2014) Isn't it the Timing for Physics Guidelines on High Dose Rate (HDR) Brachytherapy for Localized Prostate Cancer?. Int J Cancer Clin Res 1:002e. 10.23937/2378-3419/1/1/1002
Copyright: © 2014 Kim Y. This is an open-access article distributed under the terms of the Creative Commons Attribution License, which permits unrestricted use, distribution, and reproduction in any medium, provided the original author and source are credited.
Brachytherapy for Localized Prostate Cancer in Comparison with External Beam Approach
Prostate cancer has been known as the most frequently occurring (233,000 estimated new cases in 2014) and the secondly death-causing (29,480 estimated deaths in 2014) for men in the United States of America[1]. Based on the extension of tumor, prostate cancer is staged in the Tumor-Node-Metastasis (TNM) scoring system [2]. Depending upon clinical TNM staging, prostate-specific antigen (PSA) level (ng/mL) and pathologic biopsy denoted as Gleason sum score (GSS), localized prostate cancer patients are categorized into three risk groups according to the National Comprehensive Cancer Network (NCCN) guideline [3]: lowrisk (localized within half of prostate (T1-T2a), GSS < 6 and PSA < 10); intermediaterisk (spreading to entire prostate (T2b-T2c) and/or GSS7 and/or PSA 10-20); high risk (extra-capsular extension(T3a) or GSS 8-10 or PSA > 20).Seminal vesicles involvement and extension to neighboring organs is considered as very high risk [3]. Different from other malignancy, dose escalation to the prostate is highly effective, enabling local tumor control feasible even for high risk patient [4,5]. For low risk patients, several treatment options have been available with high tumor control such as androgen deprivation therapy with radiation therapy (RT), surgery (radical prostatectomy), RT (external beam or brachytherapy), chemotherapy with RT, or cryotherapy. For intermediate and high risk patients, combination of more than two treatment options is recommended [6].
Each RT technique has its pros and cons. A typical approach is external beam RT (EBRT) using photon or proton beam and it is non-invasive procedure. The modern EBRT technique has been evolved from three-dimensional conformal radiation therapy (3D CRT) and intensity modulated radiation therapy (IMRT) to stereotactic body radiation therapy (SBRT) and proton therapy. The standard fractionation scheme is 1.8-2 Gy daily dose for more than 7 weeks with image guidance. The dose distribution with IMRT technique with daily image guidance is more conformal to the target while sparing organs at risk (OARs) than that with 3D CRT, resulting in better tumor control and less toxicity. With advanced immobilization technique and cutting-edge imaging guidance system, SBRT can deliver a highly conformal dose with high daily dose and thus entire course of treatment can finish within a single week, for instance, total of 35 Gy with 7 Gy daily fraction. Due to the benefit from Bragg peak property in depth-dose curve, proton beam can spare more normal tissue than photon beam with full target coverage in spite of limited number of beams (mostly two beams for prostate cancer) used. However, all these EBRT techniques require a margin for tumor motion and setup error because radiation comes from outside of patient. Perfect patient’s motion control cannot be guaranteed and there is always a margin necessary for set-up error in positioning the patient on treatment table even if highly advanced technologies in patient immobilization, image guidance and delivery system are used. The planning target volume (PTV) margin for prostate cancer treatment planning requires as small as 5mm with which part of rectum and bladder are overlapped. Therefore, dose escalation to the prostate only with EBRT technique is always difficult because of this PTV margin, increasing toxicity of neighboring OARs.
In contrast, though it is invasive procedure, brachytherapy introduces radioactive material directly into the prostate and the radiation source moves together with the prostate during radiation delivery. Hence, brachytherapy technique does not require any PTV margin for tumor motion and setup error and it could achieve dose escalation to the prostate with much less dose to proximal OARs than EBRT technique. In low dose rate (LDR) brachytherapy, 50 - 150 radioactive seeds are implanted permanently into the prostate under real-time trans rectal ultra sound (TRUS) guidance. In High Dose Rate (HDR) brachy therapy, a single 192Ir source temporarily stays in each dwell position with corresponding optimal dwell time.
Difference in Physics Principles between EBRT and Brachytherapy
The basic physics principle to describe dose change (depth-dose curve) in patient significantly differs between EBRT and brachytherapy. Note that the discussion about proton beam is excluded. There are two major factors affecting depth-dose curve. The one is scattering and attenuation by the medium, depending upon attenuation and absorption coefficient (m and men) of the medium for the particular energy of photon beam. The other is inverse-square law, depending upon the geometrical distance (SPD) between radiation source and dose point of interest. In brachytherapy, the most dominant factor is the inverse-square law because the SPD is just order of a few centimeters. For instance, the dose at 4 cm from the source is 4 times (400%) lower than that at 2 cm if tissue scattering/attenuation is ignored. The energy of brachytherapy source is < 50 keV for LDR permanent implant (125I, 103Pd and 131Cs) and mean energy of 192Ir source is 380 keV for HDR temporary implant. The corresponding tissue scattering/attenuation over 2 cm tissue medium is < 40% (LDR) and ~ 20% (HDR), respectively.
In EBRT, the SPD is ~ 100 cm and inverse-square law effect is not as significant as in brachytherapy. For example, the dose reduction is only 4% due to inverse-square law between SPD distance of 100 cm and 102 cm. Tissue attention is the primary factor to govern exponential dose reduction for photon beam attenuation beyond the maximum dose depth (dmax). The dmax varies depending upon the energy of photon beam: 1.5, 2.5 and 3.2 cm for typical 6X, 10X and 18X photon beams clinically used. The tissue attenuation over 2 cm medium ranges from 7% to 4% reduction for typical clinical 6X to 18X photon beams. In order to guarantee the full dose coverage of the deeply located lesion (i.e, prostate cancer) while sparing OARs, multiple beam arrangement (at least, 7 beams for 6X and 5 beams for 18X) is required in EBRT. In general, a clinically acceptable plan with EBRT has homogeneous dose distribution over the prostate volume with maximum dose of < 110% of the prescribed dose. In contrast, brachytherapy brachytherapy results in very heterogeneous dose distribution within the prostate volume: 30-40% of prostate volume receives at least 150% of the prescribed dose and even more than 200% of the prescribed dose is delivered to some part of the prostate (~ 10%) in typical clinical HDR prostate brachytherapy. Due to dominant impact of inverse-square law in brachytherapy compared to EBRT (i.e, 400% vs. 4% in the example above), the dose falloff beyond the prostate is significantly steeper in brachytherapy brachytherapy plan compared to EBRT plan. Therefore, clinical brachytherapy protocols require very stringent dose constraint on OARs such as rectal dose of V100 (rectal volume receiving the prescribed dose) < 2 cc for LDR permanent implant [7] and V75 < 1 cc for HDR temporary implant [8], respectively. In a clinical protocol of EBRT [9] whose prescribed dose is 79.2 Gy, the rectal dose constraint is the maximum dose of < 107%of the prescribed dose and V95< 15% of rectum volume.
Advantage of HDR over LDR
Upon the benefit from brachytherapy physics (inverse-square law), LDR prostate seed implant (PSI) technique positions loosen or stranded seeds permanently within the prostate under real-time TRUS guidance [7,10]. Sterilized loosen seeds are first loaded into cartridges in the Mick applicator and then unloaded to a designated location inside the prostate. The implant with these loosen seeds can be customized to individual shape of the prostate, but these loosen seeds are much more vulnerable to seed migration issue. Even they can move outside of the prostate. On the contrary, the implant with stranded seeds is less flexible to be customized, but it is relatively stable against seed migration issue. Because highly conformal dose distribution can be feasible with accurate visualization of prostate and needles under real-time TRUS guidance, the LDR technique has been prevailing for low risk patients. However, there are several issues in this LDR PSI. First, it is very difficult to change the seed distribution as soon as seed implantation is completed. Second, the prescribed dose is delivered over several half-lives during which unexpected seeds migration and anatomy change of prostate due to edema (swelling of prostate from surgical implant procedure) and resolution of edema are observed in clinical practice, based on the computed tomography (CT)imaging study at the time of post-implant dosimetry. Hence, the delivered dose distribution could be different from the intended dose distribution in the treatment plan to some extent. Because of this uncontrolled behavior of seeds and change of prostate anatomy after implantation, seeds are restricted to be implanted within the prostate and LDR PSI option is recommended only for low risk patients as a monotherapy. Finally, there is unnecessary radiation exposure to any staff involved in this procedure.
In order to overcome these limitations of LDR permanent implant technique, HDR temporary implant uses a computer-controlled machine called 'after loader'. Itis programmed to maneuvera single 192Ir source into the prostate through interstitial catheters implanted. The dwell positions are defined along the catheters digitized on3D treatment planning CT images and the 192Ir source driven by the after loader stays in those dwell positions for pre-calculated optimal well times. Because the HDR after loader is remotely controlled and the patient is treated alone, there is no concern about radiation dose to any staff involved. Most importantly, there is additional variable ('dwell time') to be controlled for the optimal dose distribution with HDR while the only variable to be controlled for the optimal dose distribution with LDR is seed positions. The dose distribution with HDR is determined by the combination of dwell times and corresponding dwell positions and it can be customized to the shape of prostate by optimization process. The inverse planning algorithm available in the current commercial treatment planning system can mathematically compute the optimal dwell time distribution. This 3D image-based inverse planning together with computerized HDR after loader system can result in a highly conformal dose distribution to the target, including extra-capsular extension disease and seminal vesicles invasion.
Evolution of HDR prostate Brachytherapy
Over two decades, HDR brachytherapy technique for localized prostate cancer treatment has been clinically matured in terms of TRUS guided implant technique, 3D image-based treatment planning, and computerized after loader delivery. With these advancements of HDR technique, dose escalation has been demonstrated with excellent local control and low toxicity even for high risk patients. Hence, the clinical role of HDR prostate brachytherapy has evolved as a boost in conjunction with EBRT for low or intermediate risk patients to become effective even for high risk patients. As a monotherapy it has showed good disease control with favorable toxicity for low and intermediate risk patients. Also, it could be feasible for clinically difficult cases such as large prostate ( > 60 cc), extra-capsular extension, post-transurethral resection of prostate. Additionally, it has been considered as a salvage treatment option for locally recurrent prostate cancer. Due to the nature of radiation biology (low α/ß ratio) of prostate cancer, hypofractionation scheme was initially introduced as low as 5 Gy x 3 fractions as a boost. A lot of different hypofractionation schemes have been employed depending upon each institution's preference and the trend has evolved to deliver fewer fractions with a larger dose per fraction. For instance, ultra high single fraction dose has been delivered, i.e, 15 Gy as a boost; 19 Gy as a monotherapy.
While two orthogonal radiography films were used for treatment planning two decades ago, 3D CT image-based treatment planning with inverse planning has been routinely performed in the current clinical practice. In some academic centers, magnetic resonance imaging (MRI) and MR spectroscopy imaging are fused with planning CT images to define dominant prostatic lesion (DIL) within the prostate and to escalate DIL dose for dose painting. In order to eliminate patient transfer for CT scan and overnight hospitalization, a single fraction delivery for a single implant has been feasible using TRUS image based planning. In summary, 3D image-based HDR prostate brachytherapy has been matured, evolving and increasingly performed as a different role in various fractionation schemes.
Need for Physics Guideline on 3D Image-Based HDR Prostate Brachytherapy
With the maturity of the technique and high local tumor control with low toxicity, the use of HDR prostate brachytherapy has been increasing. Following the growing clinical interest and concern regarding this evolving technique, clinicians have been already providing guidelines from their perspectives. First, European society, GroupeEuropen de Curietherapie (GEC) and the European SocieTy for Radiotherapy & Oncology (ESTRO), published their recommendation [11] in 2005 and it was recently updated [12] (published in 2013). American Brachytherapy Society (ABS) also provided its clinical consensus guidelines [4] (2012) and appropriateness criteria [5] (2014).
However, the physics/dosimetry section in these clinician's guidelines has been a lack of details for clinical medical physicists to safely implement the technique in the individual clinic. For instance, there is no specific guideline regarding how to validate reconstructed CT or TRUS images in order to accurately define the tip of interstitial catheters for 3D image-based treatment planning. In addition, catheter displacement is a critical issue between treatment planning and delivery. It should be accurately measured using possible 3D imaging modality and corrected prior to each fraction. However, there is no detail physics guideline addressing this catheters displacement issue.
The American Association of Physicists in Medicine (AAPM) is the organization to provide guidelines and recommendations from physicists perspectives for clinical medical physicists to safely implement a clinical/technical procedure performed in the radiation oncology. So far, AAPM provided several task group (TG)reports regarding HDR brachytherapy: TG 41 [13] (1993) for afterloader technology, TG 56 [14] (1997) for code of practice regarding HDR unit and HDR treatment planning/evaluation, and TG 59 [15] (1998) for safe delivery of HDR brachytherapy. TG 40 [16] (1993) partly discussed brachytherapy treatment planning QA and TG 53 [17] (1998) included brachytherapy dose calculation commissioning. All these reports addressed the issues in 2D image-based HDR brachytherapy. Though they can be still useful to provide conceptual framework in modern 3D image-based brachytherapy, they should be updated to handle 3D issues which are different depending upon a specific technique. A newly formed TG 236 will address 3D image-based HDR planning/dosimetry and quality management issues by focusing intracavitary technique. It is noted that the scope of TG 236 will not include HDR prostate interstitial brachytherapy. For permanent prostate seed implant technique, the 3D image-based treatment planning and dosimetry issues were addressed in TG 64 [10] (1999) and image timing for post-seed implant and dose prescription and report standards was defined in TG 137 [7] (2009). The uncertainty in HDR brachytherapy procedures was discussed in TG 56 [14] (1997) and uncertainty analysis formalism was presented in TG 138 [18] (2011).
Hence, it is great timing that AAPM has to provide physics guidelines on 3D image-based treatment planning and dosimetry and quality management standards for HDR prostate brachytherapy. These guidelines will be essential for safe implementation of HDR prostate interstitial brachytherapy. The suggested charges for guidelines are as follow.
i. Review of clinical workflow for HDR prostate brachytherapy from TRUS guided implant to HDR delivery
ii. Development of quality management program for commissioning and routine QA using TG 100 concept
iii Critical review and investigation of 3D image acquisition/validation for 3D image-based treatment planning
iv. Discussion of inverse planning optimization and providing practical guidelines for treatment planning of HDR prostate brachytherapy
v. Clinical review on contouring target and OARs and development of dosimetry guidelines for clinically optimal plan
vi. Investigation of catheter displacement between treatment planning and delivery and development of verification QA procedure (including possible imaging modality) prior to each fraction
vii .Review of uncertainty for HDR prostate brachytherapy and investigation of model-based dose calculation algorithm (MBDCA) in comparison with TG 43 formalism
iiiv.Review of biophysics model for HDR prostate brachytherapy and propose a simple model to compute biological dosimetrics of target and OARs for various clinical fractionation schemes and treatment options (boost in combination with EBRT vs. monotherapy)
References
-
http://www.cancer.org/acs/groups/content/@research/documents/ webcontent/acspc-042151.pdf
-
Edge S, Byrd DR, Compton CC, et al. AJCCCancer Staging Manual 7th ed. New York, NY:Springer; 2011. pp 457-468
-
http://www.nccn.org/professionals/physician_gls/pdf/prostate.pdf
-
Yamada Y, Rogers L, Demanes DJ, Morton G, Prestidge BR, et al. (2012) American Brachytherapy Society consensus guidelines for high-dose-rate prostate brachytherapy. Brachytherapy 11: 20-32.
-
Hsu IC, Yamada Y2, Assimos DG3, D'Amico AV4, Davis BJ5, et al. (2014) ACR Appropriateness Criteria high-dose-rate brachytherapy for prostate cancer. Brachytherapy 13: 27-31.
-
Hoppe R, Phillips T, Roach M. In Leibel and Phillips Textbook of Radiation Oncology 3rd ed. Philadelphia, PA: Elsevier Saunders; 2010. pp 925-986
-
Nath R, Bice WS, Butler WM, et al. AAPM recommendations on dose prescription and reporting methods for permanent interstitial brachytherapy for prostate cancer: report of Task Group 137. Med Phys 2009;36:5310-5322.
-
Hsu IC, Bae K, Shinohara K, et al. Phase II trial of combined high-dose-rate brachytherapy and external beam radiotherapy for adenocarcinoma of the prostate: preliminary results of RTOG 0321. Int J RadiatOncolBiolPhys2010;78:751-758.
-
http://www.rtog.org/ClinicalTrials/ProtocolTable/StudyDetails.aspx?study=0126
-
Yu Y, Anderson LL, Li Z, et al. Permanent prostate seed implant brachytherapy: report of the American Association of Physicists in Medicine Task Group No. 64. Med Phys 1999;26:2054-2076.
-
Kovacs G, Potter R, Loch T, et al. GEC/ESTRO-EAU recommendations on temporary brachytherapy using stepping sources for localised prostate cancer. RadiotherOncol 2005;74:137-148.
-
Hoskin PJ, Colombo A, Henry A, et al. GEC/ESTRO recommendations on high dose rate afterloading brachytherapy for localised prostate cancer: an update. Radiother Oncol;107:325-332.
-
Holt JG. AAPM Report No. 41: remote afterloading technology. Med Phys 1993;20:1761.
-
Nath R, Anderson LL, Meli JA, et al. Code of practice for brachytherapy physics: report of the AAPM Radiation Therapy Committee Task Group No. 56. American Association of Physicists in Medicine.Med Phys 1997;24:1557-1598.
-
Kubo HD, Glasgow GP, Pethel TD, et al. High dose-rate brachytherapy treatment delivery: report of the AAPM Radiation Therapy Committee Task Group No. 59. Med Phys 1998;25:375-403.
-
Kutcher GJ, Coia L, Gillin M, et al. Comprehensive QA for radiation oncology: report of AAPM Radiation Therapy Committee Task Group 40. Med Phys 1994;21:581-618.
-
Fraass B, Doppke K, Hunt M, et al. American Association of Physicists in Medicine Radiation Therapy Committee Task Group 53: quality assurance for clinical radiotherapy treatment planning. Med Phys 1998;25:1773-1829.3.
-
DeWerd LA, Ibbott GS, Meigooni AS, et al. A dosimetric uncertainty analysis for photon-emitting brachytherapy sources: report of AAPM Task Group No. 138 and GEC-ESTRO. Med Phys2011;38:782-801.
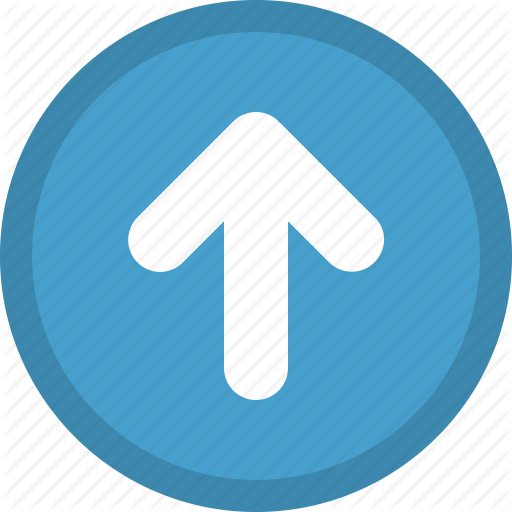