International Journal of Diabetes and Clinical Research
Involvement of Regucalcin in Lipid Metabolic Disorder and Diabetes
Masayoshi Yamaguchi
Department of Hematology and Medical Oncology, Emory University School of Medicine, Atlanta, USA
*Corresponding author:
Masayoshi Yamaguchi, PhD, Department of Hematology and Medical Oncology, Emory University School of Medicine, Room C5054, 1365 C Clifton Road NE, Atlanta, GA 30322, USA, E-mail: yamamasa1155@yahoo.co.jp
Int J Diabetes Clin Res, IJDCR-1-003, (Volume 1, Issue 1), Editorial; ISSN: 2377-3634
Received: September 29, 2014 | Accepted: October 01, 2014 | Published: October 03, 2014
Citation: Yamaguchi M (2014) Involvement of Regucalcin in Lipid Metabolic Disorder and Diabetes. Int J Diabetes Clin Res 1:003e. 10.23937/2377-3634/1410003
Copyright: © 2014 Yamaguchi M. This is an open-access article distributed under the terms of the Creative Commons Attribution License, which permits unrestricted use, distribution, and reproduction in any medium, provided the original author and source are credited.
I was much pleasured to prepare inaugural editorial for a new Journal ?International Journal of Diabetes and Clinical Research?. In this editorial, I prefer to describe an involvement of regucalcin in lipid metabolic disorder and diabetes, which is a recent topic. Regucalcin, which was discovered in 1978 [1,2], plays a multifunctional role as a suppressor protein in signal transduction in various types of cells and tissues and plays a cell physiologic role in maintaining cell homeostasis for various stimuli [3-9]. The regucalcin gene (rgn) is localized on the X chromosome and is identified in over 15 species consisting of regucalcin family [3]. Regucalcin has been demonstrated to play a multifunctional role in cell regulation of calcium homeostasis, signal transduction, protein synthesis and proteolysis, nuclear gene expression, cell proliferation and apoptosis in various types of cells and tissues including liver, kidney, heart, brain and bone [3-9]. Moreover, regucalcin was shown to play a pathophysiologic role in hyperlipidemia and diabetes. We generated regucalcin transgenic rats that reveal overexpression of endogenous regucalcin, and this animal was found to induce hyperlipidemia associated with osteoporosis [10-12]. Regucalcin was suggested to be a key molecule in lipid metabolic disorder implicated in obesity and diabetes.
Obesity and diabetes are currently a major health problem worldwide with growing in prevalence. The incidence of metabolic disease, including type 2 diabetes with obesity, is increased to epidemic levels. Obesity and diabetes induce secondary diseases with various pathophysiologic states, which are important in clinical aspects including cardiovascular disease, neural disturbance, kidney disease, osteoporosis and cancer. Obesity is based on stimulation of adipogenesis. Bone marrow mesenchymal stem cells are multipotent cells, which among other cell lineages, and give to differentiate into adipocytes, osteoblasts, chondrocytes and myoblasts [13]. This occurs through cross talk between complex signaling pathways including those derived from bone morphogenic proteins, winglesstype MMTV integration site (Wnt) proteins, hedgehogs, delta/jagged proteins, fibroblastic growth factors, insulin, insulin-like growth factors, and transcriptional regulators of adipocyte and osteoblast differentiation including peroxisome proliferators-activated receptor-gamma (PPAR?) and runt-related transcription factor 2 (Runx2) [13-15]. Insulin, which is secreted by feeding, stimulates adipogenesis from bone marrow mesenchymal stem cells. Bone marrow adiposity and mature adipocytes with obesity greatly produces tumor necrosis factor-a (TNF-α), an inflammatory cytokine [16]. This TNF-α may cause insulin resistance that leads to type 2 diabetes. Various hormones and cytokines, which include leptin, adiponectin, insulin, epinephrine, cortisol, glucagon, TNF-α and other factors, are well known as key molecules that relate to obesity and diabetes. Disturbance of these factors may play an important role in pathophysiologic conditions of obesity and diabetes.
Regucalcin was demonstrated to stimulate adipogenesis in mouse bone marrow cell culture in vitro [17], suggesting an involvement as a stimulatory factor in adipogenesis. Interestingly, exogenous regucalcin was found to suppress osteoblastogenesis and stimulate adipogenesis in mouse bone marrow culture in vitro [17,18]. Regucalcin may stimulate differentiation from bone marrow mesenchymal stem cells to adipocytes; supporting the view that regucalcin plays a regulatory role in adipogenesis. Regucalcin Transgenic (TG) rats, which overexpress endogenous regucalcin, induced a remarkable bone loss associated with increase in serum triglyceride and High-Density Lipoprotein (HDL)-cholesterol concentrations at the age of 36 weeks in vivo [10-12]. Serum free fatty acid, triglyceride, cholesterol or HDL-cholesterol concentrations were markedly increased in regucalcin TG male and female rats at 14-50 weeks of age [11]. This animal may be a useful tool in the aspects of lipid metabolic disorder and osteoporosis. Hyperlipidemia has been reported to induce in various animal models; lipoprotein lipase- deficient mice [19], low-density lipoprotein (LDL) receptor-deficient mice [20], apolipoprotein C3-KO mice [21], apolipoprotein C1 TG mice [22], very LDL lipoprotein receptor KO mice [23], cholesterol 7 alpha-hydroxylase-deficient mice [24], apoE-deficient mice [25] and hepatic myr-Akt overexpressing mice [26]. These animal models for hyperlipidemia are based on molecules that are regulated to lipid metabolism. Regucalcin is a novel protein molecule that regulates lipid metabolism [18].
Regucalcin was showed to express in the adipose tissues of normal rats [27]. Triglyceride content in the adipose tissues was increased in regucalcin TG rats with aging [27]. Liver triglyceride, total cholesterol, free fatty acid and glycogen contents were decreased in regucalcin TG rats [27]. The expression of regucalcin in the liver tissues was enhanced in regucalcin TG rats [27]. Regucalcin suppressed the activations of glycogen particulate phosphorylase a, cytoplasmic pyruvate kinase, and fructose 1,6-diphosphatase in rat liver [3]. Regucalcin may suppress glycogen synthesis in the liver and stimulate glycogenolysis in regucalcin TG rats. As the result, lipid synthesis may be stimulated in the liver tissues of the TG rats in vivo. Leptin and adiponectine are adipokines that are involved in lipid metabolism. Leptin mRNA expression in the adipose or liver tissues was found to decrease in regucalcin TG rats with aging [27]. Adiponectin mRNA expression was not changed in the adipose tissues of the TG rats, while its level was decreased in the liver tissues [27]. These decreases may be partly involved in hyperlipidemia induced in regucalcin TG rats. Thus, regucalcin may play an important role in the disorder of lipid metabolism in the liver.
Fasting induced a decrease in regucalcin mRNA expression in rat liver in vivo, and this decrease was restored after re-feeding in rats in vivo [28]. Oral administration of glucose to fasted rats caused a significant increase in hepatic regucalcin mRNA expression [28], suggesting an involvement of insulin secreted from pancreatic cells after glucose administration. Hepatic regucalcin mRNA expression was elevated administration of insulin to fasted rats in vivo [28]. Insulin was demonstrated to directly stimulate regucalcin mRNA and protein expressions in human hepatoma cells (HepG2) in vitro [29]. Thus, insulin stimulated regucalcin gene expression in liver cells. Hepatic regucalcin expression was markedly decreased after a single subcutaneous administration of streptozotocin that induces type 1 diabetes [21]. These findings support the view that regucalcin gene expression is enhanced by insulin, and that regucalcin may be involved in liver metabolic disorder related to diabetes.
Deficiency of regucalcin has been reported to cause an impairment of glucose tolerance in regucalcin knockout (KO) mice [22,30]. Regucalcin KO mice caused a significant increase in blood glucose concentration and a decrease in serum insulin levels after glucose administration compared with wild-type mice in vivo [29]. Regucalcin deficiency in mice caused an accumulation of neutral lipids and phospholipids in the liver and shortens the life span [22]. Regucalcin was not expressed in Hepatic Stellate Cells (HSCs) of both wild type and regucalcin KO mice [31,32]. Numerous HSCs was hypertrophic and contained abundant microvesicular lipid droplets in the liver cytoplasm of aged regucalcin KO mice [32]. Deficiency of regucalcin may lead to accumulation of liver lipid components.
Insulin resistance may be modeled in culture system by using cloned rat hepatoma H4-II-E cells cultured with insulin and TNF-α in vitro [33]. This in vitro model nicely mimicsed insulin resistance in human type 2 diabetic mellitus. When H4-II-E cells were cultured in the presence of TNF-α plus insulin in vitro, regucalcin was identified as an important protein, which is involved in insulin resistance, by proteome analysis [33]. Regucalcin may be a key molecule that is related to insulin resistance. Regucalcin, moreover, has been demonstrated to stimulate glucose utilization and lipid production in H4-II-E cells in vitro [34]. Overexpression of endogenous regucalcin was found to stimulate the production of triglyceride and free fatty acid in H4-II-E cells cultured with or without the supplementation of glucose in the absence of insulin [34]. Regucalcin may stimulate lipid production that is linked to glucose metabolism in liver cells in vitro. The effect of insulin, which enhances medium glucose consumption, triglyceride and free fatty acid productions in liver cells cultured with glucose supplementation, was suppressed by overexpression of regucalcin in vitro [35]. Insulin resistance in the liver is associated with the pathogenesis of Nonalcoholic Fatty Liver Disease (NAFLD). Patients with NAFLD had a significant lower level of hepatic regucalcin [36]. Hepatic regucalcin levels were decreased in a fibrosis stage-dependent manner and were correlated negatively with the homeostasis model assessment of insulin resistance, the net electronegative charge modified-LDL, and type IV collagen 7S [36]. Whether or not the decrease in hepatic regucalcin in human patients is a result or a cause of cirrhosis remains to be elucidated [36].
Regucalcin was showed to regulate the genes expression of various proteins that are related to glucose and lipid metabolism in liver cells. Overexpression of regucalcin did not reveal stimulatory effects on the gene expression of enzymes including acetyl-CoA carboxylase, HMG-CoA reductase, glucokinase and pyruvate kinase in liver cells after culture with or without glucose supplementation in the presence of insulin [34]. Overexpression of regucalcin increased the expression of Glucose Transporter 2 (GLUT 2) mRNA to enhance glucose utilization in the liver cells [34,35], and it was found to suppress the expression of Insulin Receptor (Insr) or Phosphatidylinositol 3-Kinase (PI3K) mRNAs that are an insulin signaling-related protein [34,35]. Regulatory effects of regucalcin on these gene expressions may play an important role in insulin resistance in liver cells. In addition, regucalcin may suppress signal transduction pathways that are related to insulin action in liver cells.
As described above, regucalcin may play a physiological role in lipid and glucose metabolism in the adipocytes and liver cells. Regucalcin was identified to be a molecule related to insulin resistance in liver cells. Deficiency of regucalcin impaired glucose tolerance and induces liver lipid accumulation. Overexpression of regucalcin was found to stimulate hepatic glycolysis and lipid production. Disturbance of hepatic regucalcin gene expression may lead to disorders of lipid metabolism and insulin resistance in the liver tissues, leading a hyperlipidemia. Regucalcin may play a potential pathophysiologic role as a regulatory protein implicated in lipid metabolic disorder and diabetes. Regucalcin may be a target molecule for therapy of these diseases. In this aspect, clinical studies will be expected.
Acknowledgements
The author was supported by the Foundation for Biomedical Research on Regucalcin.
References
-
Yamaguchi M, Yamamoto T (1978) Purification of calcium binding substance from soluble fraction of normal rat liver. See comment in PubMed Commons below Chem Pharm Bull (Tokyo) 26: 1915-1918.
-
Yamaguchi M (2000) Role of regucalcin in calcium signaling. See comment in PubMed Commons below Life Sci 66: 1769-1780.
-
Yamaguchi M (2011) Regucalcin and cell regulation: role as a suppressor protein in signal transduction. See comment in PubMed Commons below Mol Cell Biochem 353: 101-137.
-
Yamaguchi M (2013) Role of regucalcin in cell nuclear regulation: involvement as a transcription factor. See comment in PubMed Commons below Cell Tissue Res 354: 331-341.
-
Yamaguchi M (2013) Suppressive role of regucalcin in liver cell proliferation: involvement in carcinogenesis. See comment in PubMed Commons below Cell Prolif 46: 243-253.
-
Yamaguchi M (2013) The anti-apoptotic effect of regucalcin is mediated through multisignaling pathways. See comment in PubMed Commons below Apoptosis 18: 1145-1153.
-
Yamaguchi M (2012) Role of regucalcin in brain calcium signaling: involvement in aging. See comment in PubMed Commons below Integr Biol (Camb) 4: 825-837.
-
Yamaguchi M (2014) The role of regucalcin in bone homeostasis: involvement as a novel cytokine. See comment in PubMed Commons below Integr Biol (Camb) 6: 258-266.
-
Yamaguchi M (2014) Regulatory role of regucalcin in heart calcium signaling: Insight into cardiac failure (Review). See comment in PubMed Commons below Biomed Rep 2: 303-308.
-
Yamaguchi M, Misawa H, Uchiyama S, Morooka Y, Tsurusaki Y (2002) Role of endogenous regucalcin in bone metabolism: bone loss is induced in regucalcin transgenic rats. See comment in PubMed Commons below Int J Mol Med 10: 377-383.
-
Yamaguchi M, Igarashi A, Uchiyama S, Sawada N (2004) Hyperlipidemia is induced in regucalcin transgenic rats with increasing age. See comment in PubMed Commons below Int J Mol Med 14: 647-651.
-
Yamaguchi M (2010) Regucalcin and metabolic disorders: osteoporosis and hyperlipidemia are induced in regucalcin transgenic rats. See comment in PubMed Commons below Mol Cell Biochem 341: 119-133.
-
Muruganandan S, Roman AA, Sinal CJ (2009) Adipocyte differentiation of bone marrow-derived mesenchymal stem cells: cross talk with the osteoblastogenic program. See comment in PubMed Commons below Cell Mol Life Sci 66: 236-253.
-
Laudes M (2011) Role of WNT signalling in the determination of human mesenchymal stem cells into preadipocytes. See comment in PubMed Commons below J Mol Endocrinol 46: R65-72.
-
Kawai M, Rosen CJ (2010) PPAR?: a circadian transcription factor in adipogenesis and osteogenesis. See comment in PubMed Commons below Nat Rev Endocrinol 6: 629-636.
-
Rosen CJ, Bouxsein ML (2006) Mechanisms of disease: is osteoporosis the obesity of bone? See comment in PubMed Commons below Nat Clin Pract Rheumatol 2: 35-43.
-
Yamaguchi M, Weitzmann MN, Baile CA, Murata T (2012) Exogenous regucalcin suppresses osteoblastogenesis and stimulates adipogenesis in mouse bone marrow culture. See comment in PubMed Commons below Integr Biol (Camb) 4: 1215-1222.
-
Yamaguchi M, Murata T (2013) Involvement of regucalcin in lipid metabolism and diabetes. See comment in PubMed Commons below Metabolism 62: 1045-1051.
-
Lichtman AH, Clinton SK, Iiyama K, Connelly PW, Libby P, et al. (1999) Hyperlipidemia and atherosclerotic lesion development in LDL receptor-deficient mice fed defined semipurified diets with and without cholate. Arterioscler Thromb Vasc Biol 19: 1938-1944.
-
Jong MC, Havekes LM (2000) Insights into apolipoprotein C metabolism from transgenic and gene-targeted mice. See comment in PubMed Commons below Int J Tissue React 22: 59-66.
-
Isogai M, Kurota H, Yamaguchi M (1997) Hepatic calcium-binding protein regucalcin concentration is decreased by streptozotocin-diabetic state and ethanol ingestion in rats. Mol Cell Biochem 168: 67-72.
-
Ishigami A, Kondo Y, Nanba R, Ohsawa T, Handa S, et al. (2004) SMP30 deficiency in mice causes an accumulation of neutral lipids and phospholipids in the liver and shortens the life span. See comment in PubMed Commons below Biochem Biophys Res Commun 315: 575-580.
-
Koopmans SJ, Jong MC, Que I, Dahlmans VE, Pijl H, et al. (2001) Hyperlipidaemia is associated with increased insulin-mediated glucose metabolism, reduced fatty acid metabolism and normal blood pressure in transgenic mice overexpressing human apolipoprotein C1. Diabetologia 44: 437-443.
-
Yagyu H, Lutz EP, Kako Y, Marks S, Hu Y, et al. (2002) Very low density lipoprotein (VLDL) receptor-deficient mice have reduced lipoprotein lipase activity. Possible causes of hypertriglyceridemia and reduced body mass with VLDL receptor deficiency. J Biol Chem 277: 10037-10043.
-
Chen JY, Levy-Wilson B, Goodart S, Cooper AD (2002) Mice expressing the human CYP7A1 gene in the mouse CYP7A1 knock-out background lack induction of CYP7A1 expression by cholesterol feeding and have increased hypercholesterolemia when fed a high fat diet. See comment in PubMed Commons below J Biol Chem 277: 42588-42595.
-
Fazio S, Linton MF (2001) Mouse models of hyperlipidemia and atherosclerosis. See comment in PubMed Commons below Front Biosci 6: D515-525.
-
Yamaguchi M, Nakagawa T (2007) Change in lipid components in the adipose and liver tissues of regucalcin transgenic rats with increasing age: suppression of leptin and adiponectin gene expression. See comment in PubMed Commons below Int J Mol Med 20: 323-328.
-
Yamaguchi M, Oishi K, Isogai M (1995) Expression of hepatic calcium-binding protein regucalcin mRNA is elevated by refeeding of fasted rats: involvement of glucose, insulin and calcium as stimulating factors. See comment in PubMed Commons below Mol Cell Biochem 142: 35-41.
-
Murata T, Shinya N, Yamaguchi M (1997) Expression of calcium-binding protein regucalcin mRNA in the cloned human hepatoma cells (HepG2): stimulation by insulin. See comment in PubMed Commons below Mol Cell Biochem 175: 163-168.
-
Hasegawa G, Yamasaki M, Kadono M, Tanaka M, Asano M, et al. (2010) Senescence marker protein-30/gluconolactonase deletion worsens glucose tolerance through impairment of acute insulin secretion. See comment in PubMed Commons below Endocrinology 151: 529-536.
-
Park JK, Ki MR, Lee HR, Hong IH, Ji AR, et al. (2010) Vitamin C deficiency attenuates liver fibrosis by way of up-regulated peroxisome proliferator-activated receptor-gamma expression in senescence marker protein 30 knockout mice. Hepatology 51: 1766-1777.
-
Hong IH, Han JY, Goo MJ, Hwa SY, Ki MR, et al. (2012) Ascorbic acid deficiency accelerates aging of hepatic stellate cells with up-regulation of PPAR?. See comment in PubMed Commons below Histol Histopathol 27: 171-179.
-
Solomon SS, Buss N, Shull J, Monnier S, Majumdar G, et al. (2005) Proteome of H-411E (liver) cells exposed to insulin and tumor necrosis factor-alpha: analysis of proteins involved in insulin resistance. See comment in PubMed Commons below J Lab Clin Med 145: 275-283.
-
Nakashima C, Yamaguchi M (2006) Overexpression of regucalcin enhances glucose utilization and lipid production in cloned rat hepatoma H4-II-E cells: Involvement of insulin resistance. J Cell Biochem 99: 1582-1592.
-
Nakashima C, Yamaguchi M (2007) Overexpression of regucalcin suppresses gene expression of insulin signaling-related proteins in cloned rat hepatoma H4-II-E cells: involvement of insulin resistance. Int J Mol Med 20: 709-716.
-
Park H, Ishigami A, Shima T, Mizuno M, Maruyama N, et al. (2010) Hepatic senescence marker protein-30 is involved in the progression of nonalcoholic fatty liver disease. See comment in PubMed Commons below J Gastroenterol 45: 426-434.
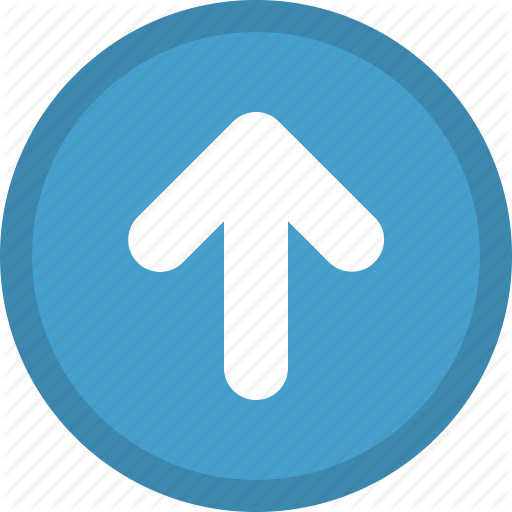