International Journal of Radiology and Imaging Technology
Radiation Protection in X-Ray Computed Tomography: Literature Review
Khalid G Alsafi*
Department of Radiology, Medical Physics Unit, King Abdul Aziz University, Saudi Arabia
*Corresponding author:
Khalid G Alsafi, Department of Radiology, Medical Physics Unit, King Abdul Aziz University, P.O. Box 80215, Jaddah 21589, Saudi Arabia, E-mail: kalsafi@kau.edu.sa
Int J Radiol Imaging Technol, IJRIT-2-016, (Volume 2, Issue 2), Review Article
Received: March 30, 2016; Accepted: July 15, 2016; Published: July 18, 2016
Citation: Alsafi KG (2016) Radiation Protection in X-Ray Computed Tomography: Literature Review. Int J Radiol Imaging Technol 2:016.
Copyright: © 2016 Alsafi KG. This is an open-access article distributed under the terms of the Creative Commons Attribution License, which permits unrestricted use, distribution, and reproduction in any medium, provided the original author and source are credited.
Abstract
The aim of this study was to evaluate radiation protection techniques in computed tomography (CT) scanning, address concerns on the increased population exposure during CT procedures, and provide a review on dose management and optimization procedures. Radiation protection in CT requires regular dose surveys and optimization of CT exposure parameters, establishing and/or implementing diagnostic references (DRLs), implementation of a comprehensive quality assurance program, reference dose levels, and CT dose saving protocols. Patient dose reduction of 40-60%, 50%, 20-30%, 20-40%, 30-50% can be achieved using tube current modulation, beam filters, thyroid and breast shields, low tube voltage for abdominal CT, automatic pitch adaptation; respectively. CT users are strongly encouraged to take advantage of these dose reduction techniques while maintaining diagnostic image quality. Current review provides updated radiation protection measures for minimizing patient radiation dose in CT without adversely affecting the quality of diagnostic information.
Keywords
Radiation protection, Computed tomography, Dose optimization strategies
Introduction
Radiation protection in CT
Radiation protection in computed tomography (CT) deserves special attention since CT is by far the largest contributor to patient radiation exposure in diagnostic radiology [1]. The United Nation Scientific Committee on Effect of Atomic Radiation (UNSCEAR) reported that on average for countries in Health-care level I, CT represents 6% of all diagnostic medical x-ray examinations but accounts for 41% of the total population radiation dose [1]. In UK, CT was reported to contribute to 47% of the collective dose from diagnostic radiology, but representing only 9% of all X-ray examinations [2,3]. Because of the technological advancement added to the clear benefit to the examined individuals, the frequency of CT examinations is increasing worldwide and the types of examination using CT are also becoming more numerous. As a result the population radiation burden is high. Many authors mention growing concerns about the long-term effects of radiation exposure during CT examinations [2,4]. It is important that these potentially very high doses be kept to a minimum through careful assessment of protocols, strict referral criteria for patients, use of automatic exposure controls and choice of scan techniques.
Dose reduction in CT should be optimized by adjustment of scan parameters (tube current, peak tube voltage and pitch) according to patient weight or age, and weight-adapted CT protocols have been suggested and published. For the purpose of minimizing radiation exposure, noisier images, if sufficient for radiological diagnosis, should be accepted. Optimized study quality also depends on region scanned and study indication. Other dose reduction strategies include restricting multiphase examination protocols, avoiding overlapping of scan regions, and only scanning the area in question. Guidelines to optimize the protection of patients during CT procedures have been provided by various international organizations [5-7]. All guidelines include reference doses that are described as diagnostic reference levels (DRLs) or guidance levels that assist in the optimization of radiation protection of the patient and permit comparisons of the performance of different CT scanners and techniques. Full optimization of CT requires implementing quality assurance program and optimization of both scanner and CT operator factors including the use of tube current modulation and other dose saving protocols.
Radiation health effects
Two primary detrimental health effects are associated with ionizing radiation: stochastic effects and deterministic effects. A stochastic effect of radiation is one in which the probability of the effect, rather than its severity, increases with radiation dose. Radiation-induced cancer and genetic effects are stochastic in nature. On contrary, deterministic effects occur when radiation dose exceeds certain threshold [8,9].
In CT examinations, the entrance skin doses are approximately 40 mGy for head examinations and approximately 20 mGy for body examinations [10]. As far as deterministic effects (tissue reactions) are concerned, ICRP notes that 'in the absorbed dose range up to around 100 mGy (low LET or high LET) no tissues are judged to express clinically relevant functional impairment [8,11]. Accordingly, deterministic effects are not expected for any patient undergoing a standard diagnostic CT examination.
Below the threshold for the induction of deterministic effects, the principal concern of any radiation exposure is the induction of stochastic (random) risks. In diagnostic radiology, these stochastic radiation risks are carcinogenesis and genetic effects that would appear in the offspring of an irradiated individual.
The principal concern for any patient undergoing a diagnostic CT examination is the risk of developing a radiation-induced cancer, which may be fatal or nonfatal. The total patient risk is related to the effective dose, which depends on the dose to each organ, organ radio sensitivity as well as patient age [12]. Children are more sensitive to radiation than adults and have a longer life expectancy. As a result, the risk for developing a radiation related cancer can be several times higher for a young child compared with an adult exposed to an identical CT scan.
At the (low) doses associated with diagnostic radiologic examinations, the radiation risk is generally taken to be proportional to the cumulative organ dose. The radiation risk from two CT scans, for example, would be approximately twice the risk of a single scan, irrespective of the time interval between the two CT scans [12].
Justification and Optimization of Protection in CT
The principles of radiation protection as stated by ICRP are justification, optimizations of protection and dose limitation [8]. Justification for examinations involving ionizing radiation, such as CT, is an important way of avoiding unnecessary exposure and thus a powerful radiation protection tool. It is widely believed that many unjustified exposures are made both in developing and industrialized countries [3,4]. Therefore, the referring physician has responsibility for the justification of an examination in individual cases and obtaining the advice of a radiologist for any alternative examination that would provide the desired information. The principle of dose limitation applies to occupational and public exposure but not for patients. On the other hand, both quality assurance (QA) and diagnostic reference dose levels (DRLs) have been recommended for implementation of the principle of optimizations of protection [8,9].
Radiation Dose Measurements in CT
In X-ray computed tomography, two quantities are proposed for expressing patient radiation dose [5,6]: weighted CTDI (CTDIw) per slice (serial scanning) or per rotation (helical scanning), and dose-length product (DLP) per complete examination.
CT dose index is defined as the quotient of the integral of absorbed dose to air along a line parallel to the axis of rotation of the scanner over a length of 100 mm and the nominal slice thickness, T [5-7]. For multi-slice scanner with Ni slices of thickness Ti,
In practice the integration range is ± 50 mm as defined by the International Electrotechnical commission (IEC) and the European guidelines in CT [5-7].
In practice is derived from the expression:
where D is the dose measured with the chamber and L is the sensitive length of the chamber (100 mm for this case). CTDI100 is expressed in terms of absorbed dose to air. It can be measured in air (CTDI100, air) or in phantom (CTDI100, phantom).
Weighted CT dose index, CTDIw
CTDIw represents the average absorbed radiation dose over the x and y directions at the center of the scan from a series of axial scans where the scatter tails are negligible beyond the 100 mm integration limit. The CTDIw is defined by the relation [5,6]:
where CTDI100,c represents the CTDI100 measured at the center of the dosimetry phantom, and CTDI100,p represents an average of measurements of CTDI100 at four different positions 10 mm below the surface of the phantom. CTDIw values can vary with nominal slice thickness, especially for the narrowest thicknesses.
Volume CT dose index, CTDIvol
To represent dose for a specific scan protocol, it is essential to take into account any gaps or overlaps between the X-ray beams from consecutive rotation of X-ray source. This is accomplished with of volume , quantity that takes into account the helical pitch or axial scan spacing, thus:
Where N is a number of simultaneously acquired tomographic slices, T is the nominal slice thickness, I is the distance moved by the patient couch per helical rotation or between consecutive scans for a series of axial scans. The quantity is known as the CT pitch factor (or pitch) for helical scanning, CTDIvol represents the average absorbed radiation dose over the x, y, and z directions. The CTDIvol provides a single CT dose parameter, based on a directly and easily measured quantity, which represents the average dose within the scan volume for a standardized (CTDI) phantom.
Dose-length product (DLP)
To better represent the overall energy delivered by a given scan protocol, the absorbed dose can be integrated along the scan length to compute the Dose-Length Product (DLP) where
DLP (mGycm) = CTDIvol (mGy) × scan length (cm) (4)
The DLP reflects the total energy absorbed (and thus the potential biological effect) attributable to the complete scan acquisition. Thus, an abdomen-only CT exam might have the same CTDIvol as an abdomen/pelvis CT exam, but the latter exam would have a greater DLP, proportional to the greater z-extent of the scan volume.
Effective dose
In diagnostic radiology, the patient effective dose, expressed in sieverts, is determined by multiplying the DLP value by a appropriate normalized coefficients which takes in to account the patient's age and specific anatomical region being imaged:
Effective dose = Conversion factor. DLP (5)
CT Parameters Affecting Patient Dose
Dose and image quality in CT generally depend on the choice of technique factors that are used to perform CT examination. The most important of the parameters that are under the control of the CT operator are as follows:
Tube voltage (kVp) determines the energy distribution of the incident x-ray beam. Variation in the tube voltage causes a substantial change in CT dose, as well as image noise and contrast. The choice of X-ray tube voltage (kVp) in CT scanning ranges from 80 to 140 kV. Increasing the X-ray tube voltage will increase the amount of radiation used in the exam, and will also increase the average photon energy. As a result, high voltages reduce image contrast, as well as reducing the amount of noise (mottle). In addition, use of high kV values may also reduce artifacts, such as beam hardening. Most of the abdominal CT examinations can be done using 120 kVp and earn 20% to 40% reduction in radiation dose compared to a value of 140 kVp. Furthermore, pediatrics CT examinations can be successfully performed using 80 kVp resulting in sufficient image quality [12,13].
Tube current/exposure time
The product of the X-ray tube current (mA) and scan time (s) is known as the mAs, which is a measure of the amount of radiation that is used to generate any radiographic or CT image. Because pediatric patients are smaller, and therefore easier to penetrate, the CT mAs used to scan pediatric patients is generally reduced relative to those used for adults [12-14].
Beam collimation and slice width
Beam collimation and slice width are related to the detector configuration used for MDCT scanning. Generally, wider x-ray beam widths result in more dose-efficient examinations, as over-beaming constitutes a smaller proportion of the detected X-ray beam. However, a wider beam width can limit the thinnest reconstructed sections for MDCT systems with less than 16 data channels. On such systems, narrow beam widths decrease dose efficiency owing to over-beaming, but are needed to allow reconstruction of thinner slice widths. Hence, beam width must be carefully selected to address the specific clinical requirements [15].
Over-beaming: is when the X-ray beam incident on the patient extends beyond the active detector area and hence part of the beam is not used for imaging purposes. Pre-patient control of x-ray tube focal spot motion and beam collimation improves scanner dose efficiency and thus reduces radiation dose. This technique reduces over-beaming by measuring the position of the beam every few milliseconds and repositioning the collimating aperture as necessary. This allows a narrower dose profile compared to systems with no focal spot tracking [15].
Over-hanging
Is the increase in dose-length product due to the additional rotation (s) required for the spiral interpolation algorithm. For MDCT scanners, the number of additional rotations is strongly dependent on pitch, and the increase in irradiation length is typically 1.5 times the total beam width. The implications of over-ranging with regard to the air kerma-length product depend on the length of the imaged body region. For spiral scans that are short relative to the total beam width, the dose efficiency (with regard to over-ranging) will decrease. It is generally more dose efficient to use a single spiral scan than multiple spiral scans for the same anatomical coverage [15].
Image thickness
MDCT technology allows for the reconstruction of relatively narrow image widths in total scan times that are comparable with, or shorter than, in single-detector CT. The detector collimation, however, must not necessarily be identical to the thickness of the reconstructed images. Thicker images, which are less noisy, can be generated from the thinner projection data. When reformations or partial volume averaging are not of concern, thicker images should be reconstructed in order to reduce noise [15].
Filtration
X-ray filters are used in radiology for cutting off the X-rays that have lower energy and do not contribute to the image but only to the patient dose. There are studies in the literature that have investigated the use of various filters and their effect on dose reduction. According to these studies, bow-tie or beam shaping filters reduce radiation dose by 50% compared with the conventional flat filters. Software noise reduction filters is an alternative, especially in high contrast examinations such as chest CT.
CT Optimization Strategies
Tube current modulations (TCM)
The main dose saving technique is certainly the automatic tube current modulation (TCM). It adjust the mAs to compensate for different levels of attenuation of the CT scanner's x-ray beam and thus accounts for the varying attenuation of the human body along the body axis ('longitudinal') and in the transverse plane ('angular'). Angular (x, and y-axis) tube current modulation involves variation of the tube current to equalize the photon flux to the detector as the x-ray tube rotates about the patient. In longitudinal (z) modulation, the mA is modulated to provide the desired level of image quality as the attenuation between anatomic regions varies. Combined angular and longitudinal (x, y, z) mA modulation varies the mA during both rotation and longitudinal movement of the patient through the x-ray beam (i.e., anterior/posterior versus lateral and shoulders versus abdomen). reductions of up to 40-60%, depending on the type of examination and the default settings [15].
Pitch ratio
In helical CT, the pitch ratio (P) is given by the table increment distance per 360 rotation of the X-ray tube divided by the X-ray beam width. Radiation dose is inversely proportional to pitch, such that a 2-fold increase in pitch results in a 50% reduction in dose (assuming all other parameters are held constant). Increasing pitch will decrease the amount of radiation needed to cover the region indicated, usually without compromising the diagnostic quality of the scan. Increasing pitch from 1.0 to 1.375:1 decreases dose by a factor of about 27% [12-14].
Scan coverage and indication
The scan length determines the extent of the irradiated portion of the body in the z-direction and is therefore directly proportional to patient radiation exposure. The scan length should be set at the lowest value possible that will still allow for the clinical question to be answered.
With the short scan acquisition times of MDCT, there is a tendency to increase the scan length to include multiple body regions either in part or completely. This increases radiation dose to the patient. It is necessary to be aware about the dose consequences of repetitive studies, requesting examinations of inappropriate anatomy, or requesting examinations for non-medically-necessary indications [15].
Optimization of Protection in X-Ray Computed Tomography
As the medical use of X-ray imaging is clearly justified because the clear benefit that weight radiation, optimization is certainly the most important parameters to consider. In medical imaging optimization include regular dose surveys for audits, applications of DRLs and QA.
Diagnostic reference levels
In order to optimize the radiation dose delivered to patients in the course of diagnostic and/or therapeutic procedures, measured radiation dose should be compared against establishment diagnostic reference levels (DRLs). These are defined by the council of the European Union as; “dose levels in medical radio diagnostic practices for typical examinations for groups of standard-sized patients or standard phantoms for broadly defined types of equipment [16]. These levels are expected not to be exceeded for standard procedures when good and normal practice regarding diagnostic and technical performance is applied.
Radiation dose in CT can be reduced as well as in other X-ray procedures using reference dose levels. When these levels are routinely exceeded, sites should initiate investigation of the appropriateness of their examination protocol to more appropriate optimize examination quality and safety. Established International DRLs are presented in table 1 (for adults) and table 2 (for children).
Table 1: Published adult DRLs for CTDIvol (mGy) and DLP (mGy•cm).
View Table 1
Table 2: Published pediatrics DRLs in belgium, canada and australia [20-22].
View Table 2
Quality assurance
QA is powerful tool for optimizations of equipment performance. The World Health Organization (WHO) [23] definition of QA in diagnostic radiology implies the optimum quality of the entire diagnostic process, i.e. the consistent production of adequate diagnostic information with minimum exposure of patients and personnel. According to WHO, quality control (QC) as applied to a diagnostic procedure covers monitoring, evaluation and maintenance. In QC of imaging equipment, a distinction is made between acceptance, status and constancy tests.
Acceptance tests are carried out after installation of new equipment or major modifications of apparatus in use [24]. The aim of acceptance tests is to demonstrate the validity of the specifications provided by the supplier and compliance with general requirements. Status tests have the same aims as acceptance tests but refer to existing installations. Constancy tests concern relatively simple measurements of a limited number of relevant parameters which show that no major changes occur in the proper functioning of the equipment. In table 3, Annual QC tests and tolerances levels for X-ray CT are presented [25].
Table 3: Annual QC tests and tolerances levels for X-ray CT.
View Table 3
Optimizations of pediatric CT
Radiation exposure in computed tomography is of concern in both adults and children. However, there are unique considerations in children since they have a higher average risk of developing cancer compared with adults receiving the same dose, the longer life expectancy in children allows more time for any harmful effects of radiation to manifest, and developing organs and tissues are more sensitive to the effects of radiation. As a result, the risk for developing a radiation-related cancer can be several times higher for a young child compared with an adult exposed to an identical CT scan. To limit radiation dose in pediatrics CT, various optimization strategies could be implemented, which might include but not limited to [26]:
• Limiting the region of coverage
• Adjusting individual CT settings based on indication, region imaged, and size of the child.
• Use pediatric protocols based on the age, weight, height, and indications to avoid over exposure.
• Significant decreases in dose can be achieved with lower kVp selections e.g. decreasing the kilovoltage to 80 or 100 kVp for smaller patients while using tube current modulation
• The small size of a child may require thinner CT slices compared with adults in order to improve spatial resolution.
Shielding considerations
In addition to the shielding that the X-ray unit assembly itself provides to the parts of the body which are not to be imaged, some patient organs like gonads, breast, thyroid and eyes within or adjacent to the primary X-ray beam can be shielded with leaded-impregnated materials placed over them and whenever possible, surrounding them. The use of thyroid and breast shields was report to decrease surface dose to the breast and thyroid by approximately 20% to 30% [27].
Conclusions
In radiology, it is normal practice to modify radiographic techniques to take into account patient characteristics, as well as the diagnostic task at hand. With increasing contributions from CT to the collective dose from medical exposure, it is important for each centre to employ certain dose reduction techniques for optimisation of radiation protection. Table 4 summarizes the dose reduction techniques used in computed tomography. We strongly encourage users to take advantage of these technical mechanisms for reducing radiation dose while maintaining diagnostic image quality.
Table 4: Selected dose optimization strategies with their expected Percentage of dose reduction.
View Table 4
References
-
Sources and effects of ionizing radiation (2000) United Nations Scientific Committee on Effect of Atomic Radiation. Report to the General Assembly with Scientific Annex, United Nations.
-
Shrimpton PC, Hillier MC, Lewis MA, Dunn M (2006) National survey of doses from CT in the UK: 2003. Br J Radiol 79: 968-980.
-
Suliman II, Abdalla SE, Ahmed NA, Galal MA, Isam Salih (2011) Survey of computed tomography technique and radiation dose in Sudanese hospitals. Eur J Radiol 80: e544-e551.
-
Vassileva J, Rehani M, Applegate k, Ahmed NA, Humoud Al-Dhuhli, et al. (2013) IAEA survey of paediatric computed tomography practice in 40 countries in Asia, Europe, Latin America and Africa: procedures and protocols. Eur Radiol 23: 623-631.
-
European guidelines on quality criteria for computed tomography. EUR 16262, European Communities.
-
Medical electrical equipment-Part 2-44: Particular requirements for the safety of X-ray equipment for computed tomography (2002) International Electrotecnical Commission, IEC-60601-2-44, IEC, Geneva.
-
The measurements, reporting and management of radiation dose in CT (2008) American Association for Physicist in Medicine, AAPM Report 96.
-
(2007) The 2007 Recommendations of the International Commission on Radiological Protection. ICRP Publication 103. Ann ICRP 37: 1-332.
-
IAEA Safety Standards for protecting people and the environment. Radiation Protection and Safety of Radiation Sources: International Basic Safety Standards (Interim edition) General Safety Requirements Part 3, No. GSR Part 3 (Interim).
-
McNitt-Gray MF (2002) AAPM/RSNA physics tutorial for residents: topics in CT: radiation dose in CT. Radiographics 22: 1541-1553.
-
AD Wrixon (2008) New ICRP recommendations. J Radiol Prot 28: 161-168.
-
Walter Huda (2007) Radiation Doses and Risks in Chest Computed Tomography Examinations. Proc Am Thorac Soc 4: 316-320.
-
Tsapaki V, Rehani M (2007) Dose management in CT facility. Biomed Imaging Interv J 3: e43.
-
Ravenel JG, Scalzetti EM, Huda W, Garrisi W (2001) Radiation exposure and image quality in chest CT examinations. AJR Am J Roentgenol 177: 279-284.
-
Managing Patient Dose in Multi-Detector Computed Tomography (MDCT) (2007) International Commission on Radiological Protection. ICRP Publication 102.
-
Bongartz G, Golding S, Jurik A (2004) European guidelines for multislice computed tomography. Luxembourg: European Commission & The Office for Official Publications of the European Communities.
-
(2002) The Swedish Radiation Protection Authority's Regulations and general advice on diagnostic standard doses and reference levels within medical x-ray diagnostics. Swedish Radiation Protection Authority
-
(2008) ACR practice guideline for diagnostic reference levels in medical x-ray imaging. In: American College of Radiology. Practice guidelines and technical standards. American College of Radiology. Reston, Va, 799-804.
-
Treier R, Aroua A, Verdun FR, Samara E, Stuessi A, et al. (2010) Patient doses in CT examinations in Switzerland: implementation of national diagnostic reference levels. Radiat Prot Dosimetry 142: 244-254.
-
Pages J, Buls N, Osteaux M (2003) CT doses in children: a multicentre study. Br J Radiol 76: 803-811.
-
Fujii K, Aoyama T, Koyama S, Kawaura C (2007) Comparative evaluation of organ and effective doses for paediatric patients with those for adults in chest and abdominal CT examinations. Br J Radio 80: 657-667.
-
Australian Radiation Protection and Nuclear Safety Agency (2011) National Diagnostic Reference Level Fact Sheet.
-
Quality Assurance in Diagnostic Radiology (1982) World Health Organization, Geneva.
-
Assurance of the quality in the diagnostic imaging department (2001) British Institute of Radiology, ISBN 0-905749-48-0.
-
Quality Assurance for CT Systems (2012) IAEA Human Health Series no 19, International Atomic Energy Agency.
-
Boone JM, Geraghty EM, Seibert JA, Wootton-Gorges SL (2003) Dose reduction in pediatrics CT: A rational approach. Radiology 228: 352-360.
-
Steven Birnbaum (2008) Radiation Safety in the Era of Helical CT: A Patient-Based Protection Program Currently in Place in Two Community Hospitals in New Hampshire. Journal of the American College of Radiology: JACR 5: 714-718.
-
McCollough CH, Zink FE, Kofler JM (2002) Dose optimization in CT: creation, implementation and clinical acceptance of size-based technique charts. Radiology 225: 591.
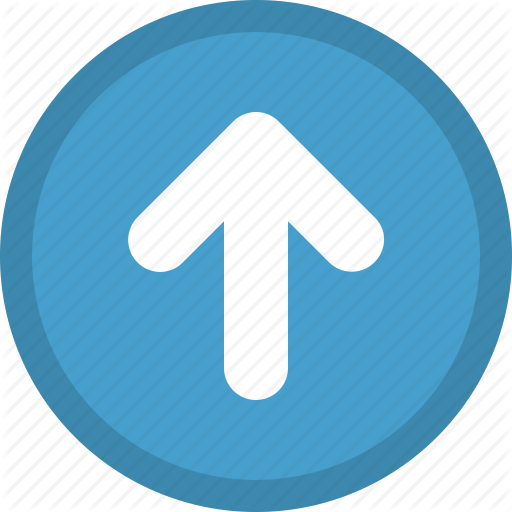