International Journal of Respiratory and Pulmonary Medicine
Inhaler Technology
D Gumani1*, William Newmarch2, Angelica Puopolo2, and Brian Casserly3
1Department of Internal Medicine, University of Limerick, Ireland
2Graduate Entry Medical School University of Limerick, Ireland
3Respiratory Division, University Hospital Limerick, Ireland
*Corresponding author: Gumani D, Department of Internal Medicine, University of Limerick, Ireland, E-mail: drdgumani@yahoo.co.uk
Int J Respir Pulm Med, IJRPM-3-064, (Volume 3, Issue 4), Review Article; ISSN: 2378-3516
Received: October 30, 2016 | Accepted: December 08, 2016 | Published: December 12, 2016
Citation: Gumani D, Newmarch W, Puopolo A, Casserly B (2016) Inhaler Technology. Int J Respir Pulm Med 3:064. 10.23937/2378-3516/1410064
Copyright: © 2016 Gumani D, et al. This is an open-access article distributed under the terms of the Creative Commons Attribution License, which permits unrestricted use, distribution, and reproduction in any medium, provided the original author and source are credited.
Introduction
Inhalers are a commonly used device to deliver medication to individuals with airway disease. With correct use, inhalers are very effective at providing quick and effective symptomatic relief within the lung, avoiding high levels of systemic exposure. These pharmacokinetic characteristics widen the therapeutic index, thus allowing for maximal therapeutic benefit with minimal adverse effects. However, this theoretical advantage is somewhat attenuated by incorrect use of the inhaler device. Improper usage of inhalers facilitates oropharyngeal deposition which significantly increases systemic absorption of the drug resulting in increased toxicity. For example, when particles that are too large are inhaled, the upper throat deposition of corticosteroids causes hoarseness and oropharyngeal candidiasis; by contrast, increased alveolar deposition of much smaller particles can cause an increase in systemic adverse drug reactions.
Inhalers, therefore, play a crucial role in the management of patients with airway disease and it is being recognised that the choice of the inhalation device appears to be as important as that of the drug molecule. Indeed in daily clinical practice, pulmonologists usually focus on the pharmacological properties of the various respiratory drugs in selecting the best possible therapeutic option, but little consideration is given to the features of the different inhalers and to the ability of the patient to use the device itself. In fact, it is often underappreciated how incorrect inhaler usage by patients is directly associated with increased healthcare resource utilisation [1].
Furthermore, with increasingly constrained societal healthcare budgets and expiring patent protection for many branded medications, there is emphasis on the development of generic inhaled drugs that are therapeutically equivalent to the original registered products, but may differ in their formulation and the design of the inhalation device. This growing scenario poses practical clinical questions about the potential impact of switching from branded to generic inhaler products in managing patients with airway disease. Indeed, the estimated 10 new drug product launches in COPD over the next 2 years will lead to even more confusion in respiratory prescribing, as there are currently more than 250 inhaler products in pharmaceutical formularies and direction is greatly needed to help healthcare professionals advise and prescribe the 'appropriate' inhaled drug/device product for their patients [1].
This article aims to provide the importance of the inhaler device in the management and treatment of patients with airway disease, and specifically to provide information to support decisions on the appropriate choice of device by healthcare professionals [2-4]. It also addresses and contrasts the advantages and limitations of different available inhalation device options.
Principles of Operation
A pneumatic nebuliser requires a pressurised gas supply as the driving force for liquid atomisation; the compressed gas is delivered through a high velocity jet which creates an area of negative pressure at the periphery of the jet stream (also called the Venturi effect). The solution in the reservoir is introduced into the gas stream and is sheared into an unstable liquid film which breaks into droplets due to the surface tension forces. Therefore, a baffle is placed in the aerosol stream which produces smaller particles causing larger particles to return to the reservoir. The aerosol produced is then delivered to the patient.
The degree of size variability between particles in an aerosol is vital for evaluating inhaler devices. This is either assessed using the mass median aerodynamic diameter (MMAD), which is the particle size (mass) below which 50% of the particle population lies, or the fine particle fraction (FPF), which is the fraction of the total drug dose < 5.8 μm in aerodynamic diameter [5]. The key characteristic of an aerosol is the particle size, with the ideal diameter falling between 1 and 5 μm with a narrow monomodal distribution. While the MMAD locates the central point of the particle size distribution, the geometric standard deviation (GSD) describes the spread of the particle size distribution, both of which are important in assessing the particle output from an inhaler device [6].
The Andersen Cascade Impactor (ACI) is the standard technique used to assess aerosolized particle size and distribution emitted from an inhaler device [7]. The ACI is an 8-stage cascade impactor with the stages typically assembled in order of decreasing particle size [8]. This allows the device to collect samples in a graduated manner. Each stage consists of a series of jets that direct any aerosolized particles towards the surface of a collection plate. If a particle has adequate inertia, which is directly related to its aerodynamic size, it will impact on the collection plate, otherwise it will remain trapped in the airstream and pass to the next stage to repeat this process [8]. The smallest particles are trapped by a final filter. This is a relatively primitive technique that cannot account for the effect of varying time constants within the lung of patients with significant airway disease. These uneven time constants will result in variable distribution throughout the lungs and the issue of deposition is further complicated by the presence of secretions and mucus that will block the passage of aerosols through the lung. An ACI is illustrated in figure 1.
The tagging of radioisotopes to the particles does allow for better in-vivo estimation via scintigraphy, but this is typically only completed in healthy volunteers and this technique is not applicable to drugs adherent to lactose carriers (i.e., most dry powder inhalers) [9]. Adequate assessment of dry powder inhaler efficacy is difficult to undertake since the lactose carrier prevents radioisotope attachment in scintigraphy testing. The lung bioavailability of inhaled medications is estimated by asking healthy volunteers to ingest activated charcoal which blocks oropharyngeal absorption therefore eliminating systemic drug absorption via this route. What these studies have shown is that lung bioavailability is significantly decreased with improper technique of inhalers, which can reduce lung deposition to as little as 5 percent from an average of 20 percent [10-16]. This is highlighted in figure 2.
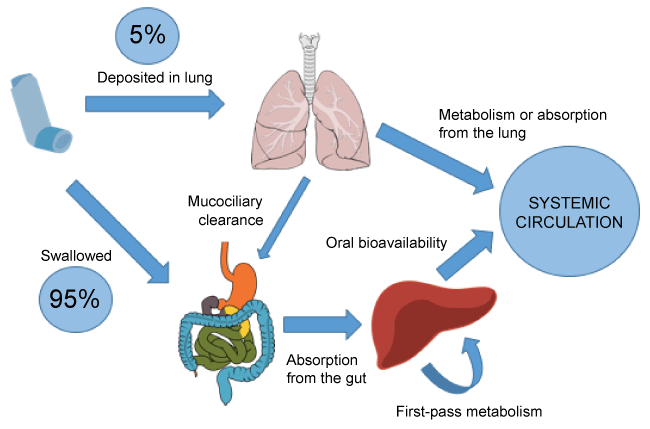
.
Figure 2: Lung bioavailability of an inhaled medication with a poor inspiratory effort.
View Figure 2
Types of Inhaler Devices
Inhaler technology is currently used to administer an extensive list of medications, including glucocorticoids, beta-agonists, anticholinergics, certain antibiotics and even insulin, directly to the lungs. And even though new inhaler technology is continuously being developed, currently available inhaler devices can be largely classified as belonging to one of three main categories: the dry powder inhaler (DPI), the metered dose inhaler (MDI), and the nebulizer.
Dry powder inhalers
DPIs were developed to provide an alternate solution to MDIs in the management of airway disease. DPIs are composed of a dry powder suspension that becomes fluid on inhalation [17]. These specialized inhalers require drug micronization and combination with a carrier particle to achieve adequate therapeutic benefit [18]. The process of micronization produces a particle with a MMAD of less than 5 μm that readily deposits in the lungs [19]. Due to the large cohesive forces that exist between such fine particles, disaggregation is difficult and requires a large carrier particle such as lactose to assist in particle separation [17,18]. As the patient inspires, turbulent forces convert the dry powder formulation to a fluid that enters the airway [17]. Finally, to achieve deposition in the lungs, a large inspiratory flow rate is required to separate the drug particles from the lactose carrier [9,17,18].
The inspiratory-dependent technology of DPIs eliminates synchronization between inspiration and inhaler administration that is required with traditional MDIs [20]. This is advantageous as it assists in minimizing the oropharyngeal deposition that occurs with improper coordination of MDIs [21]. Additionally, DPIs are a most sustainable device as they do not contain propellants and operate at lower states of energy which decreases reactions occurring between the inhaler chemicals and extrinsic surfaces [17]. Although DPIs have the potential to be advantageous in certain scenarios, the requirement of a large inspiratory flow rate (between 30 to 60 L/min) to assist in drug activation limits the use of DPIs to patients with adequate pulmonary function [18-20,22].
Future studies are currently examining the possibility of reducing the dependence of DPIs on inspiratory flow rate which can potentially allow DPIs to be very effective devices in the management of airway disease [17,18,23]. However, it should be noted that the idea of a low resistance device may be misguided because a certain amount of resistance is inherently required to ensure disaggregation of the drug from the lactose carrier; therefore, oropharyngeal deposition may occur because of the inadequate disaggregation [23,24].
Metered dose inhalers
MDIs can be further classified as either a pressurized (contains propellant) or a non-pressurized (does not contain propellant) device.
Pressurized metered dose inhalers: Developed in 1956, the pressurized metered dose inhaler (pMDI) was the first type of inhaler device to be marketed for the treatment of airway disease. It is both the most cost-effective type of inhaler device and the most widely used [25]. Basically, a pMDI device consists of a metering valve and stem, a mouthpiece actuator, and a pressurized canister containing a medication suspended within a propellant [26]. The propellant is a liquefied compressed gas that can maintain a constant vapour pressure, which is essential for proper device functionality as it allows for a consistent suspension pressure regardless of the volume remaining in the canister. Otherwise, the pressure would change with each use of the inhaler, thus severely limiting the effectiveness of the device [26].
Initially, pMDI devices contained chlorofluorocarbons (CFCs) as a propellant, but they have since been replaced by the CFC-free propellant hydrofluoroalkane (HFA). This was following the implementation of the 1987 Montreal Protocol - an international agreement to ban CFCs due to their documented role in ozone depletion [25,27-30]. This change in propellant, however, has not negatively impacted the capability of pMDI devices to adequately deliver inhaled medications to the lungs [30-33]. Furthermore, studies have shown that some HFA devices may deliver a higher fraction of the inhaled dose when compared to similar CFC devices [27,34-36].
This finding may be explained by the tendency of HFA-containing pMDIs to produce much softer and warmer plumes, resulting in less oropharyngeal deposition [37,38]. Moreover, the warmer plume decreases the number of patients experiencing a phenomenon known as the 'cold freon' effect, which is a cold sensation felt at the back of the throat following device actuation. This is most likely a result of the forceful plume impacting on the back of a patient's throat [37]. Interestingly, when HFA-containing devices were first introduced, many patients felt that the new inhalers were not actually working properly due to the absence of this so-called 'cold-freon' effect. Patients had associated this phenomenon with the effectiveness of the inhaler, therefore proper education was required to address this misconception [37].
Another benefit of many HFA-containing pMDIs is that the active drugs are in a true solution as opposed to a micronized suspension. Therefore, the drug particles will not settle in the propellant, eliminating the need to shake the device before each use [39]. With CFC devices, shaking the device was of utmost importance as improper dispersion of the suspension could reduce the delivery of some medications by up to 50% [39-42].
Breath-actuated pMDIs: Breath-actuated pMDI (BA-pMDI) devices are a newer generation of inhaler technology designed specifically to address the issue of poor actuation-inhalation coordination and thus improve patient compliance and confidence [43,44]. Similar to a conventional pMDI, these devices contain a pressurised canister, however, a spring triggers the canister to release the dose during inhalation and automatically coordinate device actuation with patient inhalation [10]. The patient is required to inhale through the mouthpiece at a low flow rate of 22-36 L/min, which is easily achieved by most patients [10,45].
Non-pressurized metered dose inhalers: The soft mist inhaler (SMI) is a propellant-free type of MDI device that releases a soft-mist of medication. Roughly 75 percent of the aerosolized particles produced by SMIs have a MMAD ≤ 5.8 μm, while approximately 5 percent of the particles have a diameter < 1 μm [46]. This soft-mist has a lower velocity and thus remains in the air for approximately seven to ten times longer than the aerosol from a typical pMDI, significantly reducing the amount of synchronization required to successfully operate the device [47]. This results in decreased oropharyngeal deposition while also increasing the window for successful inhalation [46,48]. Furthermore, a lower nominal dose is required to achieve the same therapeutic effect as a typical pMDI [47]. Scintigraphy studies have shown that SMIs achieve greater lung and lesser oropharyngeal deposition when compared to both DPIs and pMDIs [49,50].
Nebulizers
Nebulizers are a type of inhaler device that aerosolizes a solution of medication using compressed gas, a mesh, or ultrasonic waves. Depending on patient preference, the aerosol can then be administered by a facemask, mouthpiece or potentially a nasal cannula. Presently, there are three main types of nebulizer devices: jet, mesh, and ultrasonic.
Jet nebulizer: Jet nebulizers produce an aerosol by delivering a jet flow of compressed air or oxygen through a narrow opening, creating an area of negative pressure directly above a reservoir of medication [51]. This is known as the Venturi effect - the reduction in fluid pressure that results when a fluid flows through a constriction. The resulting suction that is created pulls the solution into the gas stream, generating an aerosol [52]. The droplets generated by this primary process have a mean size that ranges widely between 14 μm and > 500 μm [53]. A baffle placed in the aerosol stream helps ensure the formation of respirable particles (1 to 10 μm), while simultaneously preventing the inhalation of larger droplets [53].
Mesh nebulizer: Mesh nebulizers are portable, battery operated devices that produce a liquid aerosol by forcing a solution of medication through a mesh or plate with multiple apertures [54]. This avoids the requirement of a compressed air source or an internal baffling system. The particle size and FPF are all functions of the exit diameter of the aperture hole (approximately 3 μm in some devices), which can be adjusted to suit different clinical applications [55]. Furthermore, these devices typically generate aerosols with a high FPF compared to conventional jet or ultrasonic nebulizers, thus improving their ability to deliver drugs to the lower respiratory tract [55].
Ultrasonic nebulizer: Ultrasonic nebulizers convert electrical energy into high-frequency ultrasonic waves using a power unit. The high-frequency ultrasonic waves then stimulate the mechanical vibration of a piezoelectric element in the transducer. This transmits the high-frequency vibrations to the surface of the liquid reservoir to create an aerosol [56]. The aerosol is then delivered to the patient using an electric fan or the patient's own inspiratory effort. The droplets produced by ultrasonic nebulizers tend to contain particles with a higher MMAD (approximately 1 to 6 μm depending on the manufacturer) than particles from a jet nebulizer [52]. Particle size is inversely proportional to the frequency of the ultrasonic waves. However, it should be noted that ultrasonic nebulizers produce a heating effect (1 to 2 °C) which may denature thermally sensitive drugs such as proteins and therefore are not suitable for nebulising some suspensions, for example DNAses [57]. Experimentally it has been shown that cooling in combination with a surfactant is one potential approach to stabilizing proteins for ultrasonic nebulization, but this also significantly reduces device output [57].
Spacers and Holding Chambers
Spacers and valved holding chambers were designed to reduce the amount of drug lost to peripheral deposition when using MDIs [19]. Both spacers and valved holding chambers function to decrease the velocity and size of drug particles prior to arrival at the mouth, which results in increased drug bioavailability and a reduction in particle loss due to external deposition [23]. Additionally, the unique design of valved holding chambers eliminates the requirement for proper coordination between patient inhalation and actuation of an MDI device [17,20]. This is advantageous as improper inhaler technique is a large contributing factor in oropharyngeal deposition. Caution must be taken with the use of spacers as exhalation into the device prior to drug inhalation can dilute the medication dose. This dilution effect is not seen with valved holding chambers due to the presence of a valve between the chamber and mouthpiece that prevents the entrance of exhaled breath into the chamber [17].
While these tools provide effective solutions to issues that arise with MDI usage, these advantages come at an additional burden to the healthcare system [20]. Their large and bulky nature also eliminates the portability of the inhaler device which is one of the main advantages of MDIs [20,23]. Additionally, the large size of a spacer or holding chamber may, to some extent, prevent the immediate use of an inhaler in a time of crisis [20].
Technology Limitations
The key issues associated with the use of inhaler devices are insufficient coordination between actuation and inhalation, oropharyngeal deposition, and to a lesser extent, poor breath-hold after inhalation.
Actuation-inhalation coordination
Inadequate coordination between actuation and inhalation is a significant cause of poor delivery of inhaled medications to the lower airways, especially in children and the elderly. This problem can be avoided through the use of a valved holding chamber as described previously or a breath-actuated DPI device. More recently, BA-pMDI devices have been designed that solve the issue of patient coordination [58]. These breath-actuated devices actually sense the patient's breath through the actuator and automatically synchronize device actuation with inhalation. Studies have shown that patients generally prefer the BA-pMDIs over the conventional devices as they find them simpler to operate [59,60].
Upper airway deposition
MDI devices generally deliver an aerosol plume faster than a patient can inhale, which is further compounded when a patient does not inhale long enough after device actuation [21]. In the case of some DPI devices, patients seldom achieve the maximum force required during inhalation to disperse the powder [38]. These issues result in a significant amount of medication being deposited in the back of the throat or the tongue, i.e., the upper airways. Oropharyngeal deposition can lead to inadequate delivery of medication to the lungs, while also increasing systemic absorption and the likelihood of unnecessary side effects such as oral thrush with inhaled glucocorticoids. Studies have shown that approximately 10 percent of the inhaled dose reaches the lungs of most patients, while 80 percent is deposited in the upper airways [10,26,34,36,38,61].
With respect to nebulizers, the breathing pattern of the patient can significantly affect the amount of aerosol deposited in the upper airways. Patients should be directed to adopt a slow breathing pattern with a normal tidal volume and an occasional deep breath to increase lower airway deposition in the lungs.
Insufficient breath-hold
It is generally accepted that a 5 to 10 second breath-hold is needed at the end of inhalation from either an MDI or a DPI to avoid substantial exhalation of the medication. This is supported by a study assessing lung deposition of HFA - and CFC-beclomethasone using varying breath-hold times that found a 16 percent reduction in lung deposition for a 1 second breath-hold when compared to a 10 second breath-hold [35].
Patient Education
Education and training in inhaler usage for both patients and healthcare professional alike, is an integral part in the management of airway disease. Issues with inhaler technique are extremely common and can lead to poor symptom control as a result. Prescribing inhalers should be done only after patients have received adequate training and have demonstrated proper usage of the inhaler. The British Thoracic Society recommends developing a personalized action plan for individuals suffering from airway disease and ensuring there are adequate trained personnel in practice to facilitate educational demonstrations [62]. Written instructions have proven to be ineffective, but rather, face-to-face or video demonstration (with the physician or with a trained nurse) is more beneficial at ensuring adequate inhaler technique and treatment adherence [63,64]. Placebo inhalers (available from inhaler manufacturers) can help with demonstrating correct inhaler technique, but these placebo devices are for single-person use only. In addition to proper demonstration, periodical follow-up and evaluation of inhaler technique has shown to be essential in ensuring correct usage [19]. Furthermore, printable handouts/flyers that provide information on inhaler technique as well as step-by-step instructions on correct usage of a respective inhaler are readily available.
Future Considerations
While never quite reaching mainstream use due to multiple technical difficulties, the experience with inhaled insulin has shown that the potential to deliver drugs systemically through the inhaled route is a real possibility. Furthermore, the incorporation of light porous particles into dry powder formulations will increase the number of antibiotics that can be delivered through an inhaler device. In the very near future, it is possible that we will be routinely treating bacterial lung infections using inhaler technology. This further emphasizes the importance of proper education to ensure maximal therapeutic benefit.
Conclusion
The advent of inhaler devices revolutionized the management of airway disease. These compact and portable devices facilitate quick therapeutic relief during exacerbations of airway disease while minimizing systemic exposure and the therapeutic dose. A lower dose of drug is often advantageous for medications with a narrow therapeutic index or for drugs which have high toxicity; delivering the medications to the site of action for a localized effect, resulting in a rapid clinical response with few side effects.
While inhalers have proven to be very effective devices, the broad range of devices has the potential to overwhelm physicians when deciphering which one is most suitable for a particular patient. While MDIs have proven to be effective at minimizing oropharyngeal deposition, inadequate coordination between actuation and inhalation decreases the efficacy of this device. Conversely, DPIs have eliminated the need for synchronization between actuation and inhalation, but drug activation requires a large inspiratory flow rate which is not always realistic in patients suffering from airway disease.
With such a diverse range of available inhaler devices, it is imperative that physicians understand not only the pharmacokinetic principles of each inhaler device, but the salient advantages and disadvantages of each device as well. Combining knowledge of inhalers with patient ability will help ensure that the patient is receiving the best therapy possible. For instance, simply being aware that a patient is unable to produce an inspiratory flow rate between 30 to 60 L/min would discourage a physician from prescribing a DPI that would eventually be proven ineffective. Additionally, having a working knowledge of other devices such as nebulizers, spacers and valved holding chambers is advantageous as these devices can be utilized to minimize the issues associated with traditional MDIs or act as an alternative option to either MDIs or DPIs. Shifting the focus of prescribing based on pharmacological principles to also include prescribing based on physiological principles of inhalers will help ensure better management and potentially minimize exacerbations of airway disease.
Inhalers have proven to be an invaluable tool in the management of airway disease. As the continued burden of inhalers on the healthcare system increases, it is imperative for both the physician and patient to continually educate themselves on the properties of inhalers and proper inhaler technique. Proper inhaler education will help to ensure that a patient is prescribed the most appropriate device to address their healthcare needs.
References
-
Bonini M, Usmani OS (2015) The importance of inhaler devices in the treatment of COPD. COPD Research and Practice 1: 9.
-
Sanchis J, Corrigan C, Levy ML, Viejo JL, ADMIT Group (2013) Inhaler devices - from theory to practice. Respir Med 107: 495-502.
-
Lavorini F, Levy ML, Dekhuijzen PN, Crompton GK, ADMIT Working Group (2009) Inhaler choice and inhalation technique: key factors for asthma control. Prim Care Respir J 18: 241-242.
-
Vincken W, Dekhuijzen PR, Barnes P, ADMIT Group (2010) The ADMIT series - Issues in inhalation therapy. 4) How to choose inhaler devices for the treatment of COPD. Prim Care Respir J 19: 10-20.
-
Newman SP, Chan HK (2008) In vitro/in vivo comparisons in pulmonary drug delivery. J Aerosol Med Pulm Drug Deliv 21: 77-84.
-
Mitchell JP, Nagel MW, Wiersema KJ, Doyle CC (2003) Aerodynamic particle size analysis of aerosols from pressurized metered-dose inhalers: comparison of Andersen 8-stage cascade impactor, next generation pharmaceutical impactor, and model 3321 Aerodynamic Particle Sizer aerosol spectrometer. AAPS PharmSciTech 4: E54.
-
Nichols SC, Mitchell JP, Shelton CM, Roberts DL (2013) Good Cascade Impactor Practice (GCIP) and considerations for "in-use" specifications. AAPS PharmSciTech 14: 375-390.
-
Copley Scientific (2016) Andersen Cascade Impactor (ACI).
-
Rahimpour Y, Hamishehkar H (2012) Lactose engineering for better performance in dry powder inhalers. Adv Pharm Bull 2: 183-187.
-
Newman SP, Weisz AW, Talaee N, Clarke SW (1991) Improvement of drug delivery with a breath actuated pressurised aerosol for patients with poor inhaler technique. Thorax 46: 712-716.
-
Pickering H, Pitcairn GR, Hirst PH, Bacon PR, Newman SP, et al. (2000) Regional lung deposition of a technetium 99m-labeled formulation of mometasone furoate administered by hydrofluoroalkane 227 metered-dose inhaler. Clin Ther 22: 1483-1493.
-
Newman SP (1993) Scintigraphic assessment of therapeutic aerosols. Crit Rev Ther Drug Carrier Syst 10: 65-109.
-
Newman S, Pitcairn G, Steed K, Harrison A, Nagel J (1999) Deposition of fenoterol from pressurized metered dose inhalers containing hydrofluoroalkanes. J Allergy Clin Immunol 104: S253-257.
-
Melchor R, Biddiscombe MF, Mak VH, Short MD, Spiro SG (1993) Lung deposition patterns of directly labelled salbutamol in normal subjects and in patients with reversible airflow obstruction. Thorax 48: 506-511.
-
Laube BL, Edwards AM, Dalby RN, Creticos PS, Norman PS (1998) The efficacy of slow versus faster inhalation of cromolyn sodium in protecting against allergen challenge in patients with asthma. J Allergy Clin Immunol 101: 475-483.
-
Hindle M, Newton DA, Chrystyn H (1993) Investigations of an optimal inhaler technique with the use of urinary salbutamol excretion as a measure of relative bioavailability to the lung. Thorax 48: 607-610.
-
Telko MJ, Hickey AJ (2005) Dry powder inhaler formulation. Respir Care 50: 1209-1227.
-
Atkins PJ (2005) Dry powder inhalers: an overview. Respir Care 50: 1304-1312.
-
Bailey W, Hess D (2016) The use of inhaler devices in adults. In: Post T, ed. UpToDate. Waltham, MA.
-
Rau JL (2006) Practical problems with aerosol therapy in COPD. Respir Care 51: 158-172.
-
Ibrahim M, Verma R, Garcia-Contreras L (2015) Inhalation drug delivery devices: technology update. Med Devices (Auckl) 8: 131-139.
-
Janssens W, VandenBrande P, Hardeman E, De Langhe E, Philps T, et al. (2008) Inspiratory flow rates at different levels of resistance in elderly COPD patients. Eur Respir J 31: 78-83.
-
Labiris NR, Dolovich MB (2003) Pulmonary drug delivery. Part II: the role of inhalant delivery devices and drug formulations in therapeutic effectiveness of aerosolized medications. Br J Clin Pharmacol 56: 600-612.
-
Feddah MR, Brown KF, Gipps EM, Davies NM (2000) In-vitro characterisation of metered dose inhaler versus dry powder inhaler glucocorticoid products: influence of inspiratory flow rates. J Pharm Pharm Sci 3: 318-324.
-
Brocklebank D, Ram F, Wright J, Barry P, Cates C, et al. (2001) Comparison of the effectiveness of inhaler devices in asthma and chronic obstructive airways disease: a systematic review of the literature. Health Technol Assess 5: 1-149.
-
Newman SP (2005) Principles of metered-dose inhaler design. Respir Care 50: 1177-1190.
-
Leach CL (2005) The CFC to HFA transition and its impact on pulmonary drug development. Respir Care 50: 1201-1208.
-
Brindley A (1999) The chlorofluorocarbon to hydrofluoroalkane transition: the effect on pressurized metered dose inhaler suspension stability. J Allergy Clin Immunol 104: S221-226.
-
Langley SJ, Sykes AP, Batty EP, Masterson CM, Woodcock A (2002) A comparison of the efficacy and tolerability of single doses of HFA 134a albuterol and CFC albuterol in mild-to-moderate asthmatic patients. Ann Allergy Asthma Immunol 88: 488-493.
-
Bronsky E, Ekholm BP, Klinger NM, Colice GL (1999) Switching patients with asthma from chlorofluorocarbon (CFC) albuterol to hydrofluoroalkane-134a (HFA) albuterol. J Asthma 36: 107-114.
-
Escribano A, Tutuncu A, Lohr I, Carlholm M, Polanowski T (2006) Clinical comparability between the CFC and HFA budesonide pressurised metered-dose inhalers in paediatric patients with asthma: a randomised controlled trial. Curr Med Res Opin 22: 1085-1092.
-
Singh D, Tutuncu A, Lohr I, Carlholm M, Polanowski T (2007) Budesonide administered using chlorofluorocarbon and hydrofluoroalkane pressurized metered-dose inhalers: pharmacokinetics, pharmacodynamics and clinical equivalence. Int J Clin Pharmacol Ther 45: 485-495.
-
Shapiro GS, Klinger NM, Ekholm BP, Colice GL (2000) Comparable bronchodilation with hydrofluoroalkane-134a (HFA) albuterol and chlorofluorocarbons-11/12 (CFC) albuterol in children with asthma. J Asthma 37: 667-675.
-
Newman S, Salmon A, Nave R, Drollmann A (2006) High lung deposition of 99mTc-labeled ciclesonide administered via HFA-MDI to patients with asthma. Respir Med 100: 375-384.
-
Leach CL, Colice GL (2010) A pilot study to assess lung deposition of HFA-beclomethasone and CFC-beclomethasone from a pressurized metered dose inhaler with and without add-on spacers and using varying breathhold times. J Aerosol Med Pulm Drug Deliv 23: 355-361.
-
Nave R, Zech K, Bethke TD (2005) Lower oropharyngeal deposition of inhaled ciclesonide via hydrofluoroalkane metered-dose inhaler compared with budesonide via chlorofluorocarbon metered-dose inhaler in healthy subjects. Eur J Clin Pharmacol 61: 203-208.
-
Gabrio BJ, Stein SW, Velasquez DJ (1999) A new method to evaluate plume characteristics of hydrofluoroalkane and chlorofluorocarbon metered dose inhalers. Int J Pharm 186: 3-12.
-
Hess DR (2008) Aerosol delivery devices in the treatment of asthma. Respir Care 53: 699-723.
-
Levy ML, Dekhuijzen P, Barnes PJ, Broeders M, Corrigan CJ, et al. (2016) Inhaler technique: facts and fantasies. A view from the Aerosol Drug Management Improvement Team (ADMIT). NPJ Prim Care Respir Med 26: 16028.
-
Thorsson L, Kenyon C, Newman SP, Borgström L (1998) Lung deposition of budesonide in asthmatics: a comparison of different formulations. Int J Pharm 168: 119-127.
-
Everard ML, Devadason SG, Summers QA, Le Souëf PN (1995) Factors affecting total and "respirable" dose delivered by a salbutamol metered dose inhaler. Thorax 50: 746-749.
-
Hatley RH, Parker J, Pritchard JN, von Hollen D (2016) Variability in Delivered Dose from Pressurized Metered-Dose Inhaler Formulations Due to a Delay Between Shake and Fire. J Aerosol Med Pulm Drug Deliv.
-
Price DB, Pearce L, Powell SR, Shirley J, Sayers MK (1999) Handling and acceptability of the Easi-Breathe device compared with a conventional metered dose inhaler by patients and practice nurses. Int J Clin Pract 53: 31-36.
-
Hampson NB, Mueller MP (1994) Reduction in patient timing errors using a breath-activated metered dose inhaler. Chest 106: 462-465.
-
Baum EA, Bryant AM (1988) The development and laboratory testing of a novel breath-actuated pressurized inhaler. J Aerosol Med 1: 219-220.
-
Dalby R, Spallek M, Voshaar T (2004) A review of the development of Respimat Soft Mist Inhaler. Int J Pharm 283: 1-9.
-
Anderson P (2006) Use of Respimat Soft Mist inhaler in COPD patients. Int J Chron Obstruct Pulmon Dis 1: 251-259.
-
Panos RJ (2013) Efficacy and safety of eco-friendly inhalers: focus on combination ipratropium bromide and albuterol in chronic obstructive pulmonary disease. Int J Chron Obstruct Pulmon Dis 8: 221-230.
-
Newman SP, Brown J, Steed KP, Reader SJ, Kladders H (1998) Lung deposition of fenoterol and flunisolide delivered using a novel device for inhaled medicines: comparison of RESPIMAT with conventional metered-dose inhalers with and without spacer devices. Chest 113: 957-963.
-
Pitcairn G, Reader S, Pavia D, Newman S (2005) Deposition of corticosteroid aerosol in the human lung by Respimat Soft Mist inhaler compared to deposition by metered dose inhaler or by Turbuhaler dry powder inhaler. J Aerosol Med 18: 264-272.
-
Lavorini F (2013) The challenge of delivering therapeutic aerosols to asthma patients. ISRN Allergy 2013: 102418.
-
Hess DR (2000) Nebulizers: principles and performance. Respir Care 45: 609-622.
-
Nerbrink O, Dahlbäck M, Hansson HC (1994) Why do medical nebulizers differ in their output and particle size characteristics? J Aerosol Med 7: 259-276.
-
Dhand R (2004) New frontiers in aerosol delivery during mechanical ventilation. Respir Care 49: 666-677.
-
Dhand R (2002) Nebulizers that use a vibrating mesh or plate with multiple apertures to generate aerosol. Respir Care 47: 1406-1416.
-
Yeo LY, Friend JR, McIntosh MP, Meeusen EN, Morton DA (2010) Ultrasonic nebulization platforms for pulmonary drug delivery. Expert Opin Drug Deliv 7: 663-679.
-
Niven RW, Ip AY, Mittelman S, Prestrelski SJ, Arakawa T (1995) Some factors associated with the ultrasonic nebulization of proteins. Pharm Res 12: 53-59.
-
Stein SW, Sheth P, Hodson PD, Myrdal PB (2014) Advances in metered dose inhaler technology: hardware development. AAPS PharmSciTech 15: 326-338.
-
Lenney J, Innes JA, Crompton GK (2000) Inappropriate inhaler use: assessment of use and patient preference of seven inhalation devices. EDICI. Respir Med 94: 496-500.
-
Chapman KR, Love L, Brubaker H (1993) A comparison of breath-actuated and conventional metered-dose inhaler inhalation techniques in elderly subjects. Chest 104: 1332-1337.
-
Darquenne C (2012) Aerosol deposition in health and disease. J Aerosol Med Pulm Drug Deliv 25: 140-147.
-
British Thoracic Society, Scottish Intercollegiate Guidelines Network (2016) British guideline on the management of asthma. Edinburgh: SIGN.
-
van der Palen J, Klein JJ, Kerkhoff AH, van Herwaarden CL, Seydel ER (1997) Evaluation of the long-term effectiveness of three instruction modes for inhaling medicines. Patient Educ Couns 32: S87-95.
-
Bosnic-Anticevich SZ, Sinha H, So S, Reddel HK (2010) Metered-dose inhaler technique: the effect of two educational interventions delivered in community pharmacy over time. J Asthma 47: 251-256.
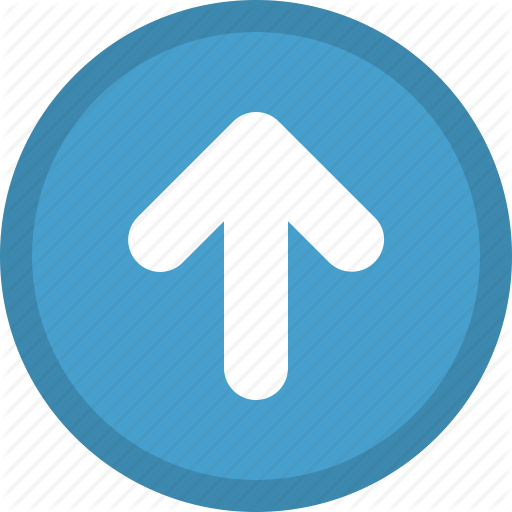