International Journal of Stem cell Research and Therapy
Stem Cells in Adult Homeostasis, Regeneration and Tissue Development of the Digestive Tract Epithelium
Valeria Fernandez Vallone and Marie-Isabelle Garcia*
Institut de Recherche Interdisciplinaire en Biologie Humaine et Moléculaire (IRIBHM), Université Libre de Bruxelles ULB, Belgium
*Corresponding author: Marie-Isabelle Garcia, Institut de Recherche Interdisciplinaire en Biologie Humaine et Moléculaire (IRIBHM), Faculty of Medicine, Université Libre de Bruxelles ULB, Route de Lennik 808, 1070, Brussels, Belgium, Tel: 32 2 555 6415, Fax: 32 2 555 4655, E-mail: mgarcia@ulb.ac.be
Int J Stem Cell Res Ther, IJSCRT-3-038, (Volume 3, Issue 2), Mini Review; ISSN: 2469-570X
Received: May 27, 2016 | Accepted: June 26, 2016 | Published: July 01, 2016
Citation: Fernandez-Vallone V, Garcia MI (2016) Stem Cells in Adult Homeostasis, Regeneration and Tissue Development of the Digestive Tract Epithelium. Int J Stem Cell Res Ther 3:038. 10.23937/2469-570X/1410038
Copyright: © 2016 Fernandez-Vallone V et al. This is an open-access article distributed under the terms of the Creative Commons Attribution License, which permits unrestricted use, distribution, and reproduction in any medium, provided the original author and source are credited.
Abstract
The gastrointestinal epithelium is one of the tissues with highest self-renewing rates under steady-state conditions, and it thereby constitutes an excellent model to better understand how tissues maintain homeostasis. In the past decade, intense research in this field has allowed identifying stem cells responsible for this task and has contributed to uncover the main molecular mechanisms associated with self-renewal and differentiation properties of these cells. One of the future challenges is to fully dissect signaling processes associated with adult regeneration to design new therapies in patients with gastro-intestinal disorders.
Here, we review recent advances regarding the identity of cells involved in adult homeostasis and regeneration in the small intestine and stomach. The recent characterization of the developmental epithelial progenitors involved in tissue morphogenesis has provided evidences that epithelial remodeling involved in fetal and adult regenerating tissues share common molecular processes.
Keywords
Digestive tract, Stem cells, Fetal progenitors, Development, Regeneration, Dedifferentiation, Organoids, Spheroids
A Complex Adult Stem Cell Pool Under Homeostasis
Small intestine
The adult intestinal epithelium is a specialized tissue devoted to nutriments absorption, which also constitutes an important barrier against pathogens or environmental toxic agents. Under homeostatic conditions, this epithelium, made of a unique architecture of crypt/villus units, constantly undergoes complete replacement in a period of time estimated between 2-5 days in the mouse. Such efficient self-renewal is ensured by active stem cells present in the bottom of the crypts that are also called Crypt base columnar cells (CBCs) and express Lgr5 as a specific marker (Figure 1 and Table 1) [1]. CBCs are both able to self-renew and differentiate into all the cell lineages of the epithelium, absorptive enterocytes, mucus-producing Goblet cells, hormones secreting enteroendocrine cells, Paneth cells generating antimicrobial products and the Type 2 immune response-inducer Tuft cells [1]. This pool of actively cycling Lgr5+ stem cells exhibits a transcriptome coherent with high canonical Wnt and Notch activities. Amongst the most common other markers, CBCs express Ascl2, Smoc2, Olfm4, Tnfrsf19 and low levels of the Sox9 and Msi1 markers [2-5]. Other populations of slowly-cycling or label-retaining (LRC) stem cells, located at the so-called "+4 position" above the Paneth cell zone, have been identified as expressing high levels of the following markers: Bmi1, Tert, Hopx, Lrig1 or Msi1 (Table 1) [5-9]. These LRCs are also referred reserve stem cells as they demonstrate low but significant long-term lineage tracing activity generating all intestinal lineages, essentially in the proximal intestine under homeostatic conditions (Figure 1). Whether the cells isolated by these various markers represent a unique or distinct stem cell populations needs to be further clarified. Indeed, cells expressing high levels of Sox9 (Sox9high) have been reported to be enriched in both Bmi1 and Hopx markers, suggesting at least some overlapping in these reserve cell types [4]. Despite some controversy about the actual existence of a quiescent stem cell pool due to detection of reserve stem cell markers in Lgr5+ CBCs and some inconsistency in some of the available reporter mouse lines, increasing evidences point to the existence of reserve cells functionally distinct from the CBCs, displaying lower levels of Wnt activity together with reduced sensitivity to this pathway and lower levels of cell-cycle related genes along with higher expression of Cdkn1a (p21) as compared to the Lgr5+ pool [2,3,10].
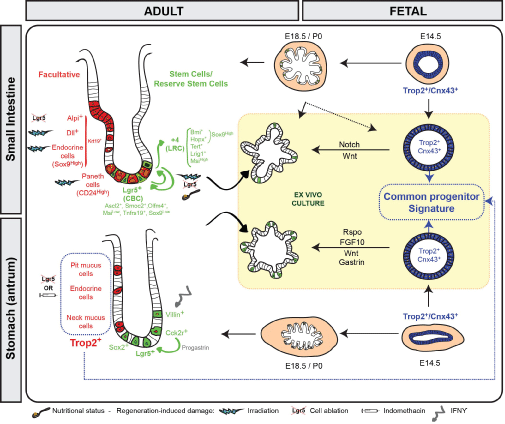
.
Figure 1: Scheme showing in vivo and parallel ex vivo maturation processes for small intestine and stomach. Fetal progenitors at embryonic stage E14.5 (Trop2+/Cnx43+), which give rise to spheroids are depicted in blue. Adult stem cells and reserve stem cells involved in homeostasis maintenance or regeneration and growing as organoids are shown in green. Facultative cells implicated as well in regeneration processes after damage are displayed in red.
View Figure 1
Table 1: Summary of fetal progenitors, adult active and reserve stem cells markers and characteristics in small intestine and stomach.
View Table 1
Stomach
The glandular stomach is organized into anatomical regions delineating two main types of glands. The proximal corpus glands contain several cell types: parietal cells producing hydrochloric acid, zymogen-secreting chief cells, hormone-generating endocrine cells as well as pit and neck cells secreting different types of mucins. The distal antral glands are mainly composed of pit, neck and endocrine cells [11]. Under homeostatic conditions, self-renewal of corpus glands is ensured by proliferative stem/progenitor cells essentially located in the central isthmus zone. Some of these progenitors have been identified as expressing TFF2 transcripts [12]. In the bottom of antral glands, Lgr5+ stem cells expressing several markers common to the small intestinal Lgr5+ stem cell pool have been demonstrated to give rise to all epithelial cell lineages (Figure 1) [13]. Although not initially reported, recent studies have evidenced the presence of Lgr5+ cells in the corpus with characteristics of terminally differentiated chief cells [14-16]. A distinct Sox2+ stem cell population has been described to be located in corpus and antral glands but its transcriptome remains to be determined [17]. Another type of actively cycling cells, located above the Lgr5+ cell zone in antral glands, expresses the Cck2r marker and contributes to constant renewal (Figure 1) [18]. This antral Cck2r+ pool likely differs from the Cck2r+ cells that label differentiated parietal and endocrine cells and some neck progenitors in the isthmus region of the corpus [19].
Identification of Epithelial Progenitors Involved in Tissue Morphogenesis
Whereas coexistence of several pools of stem cells has been reported in the adult intestinal and gastric epithelia that ensure tissue homeostasis under steady-state conditions, much less was known till recently about the identity of fetal progenitors involved in tissue morphogenesis. In the mouse, the endodermally-derived gut tube, early organized into distinct organ-specific molecular domains, condenses around embryonic stage E9 to become a pseudostratified epithelium [20]. Around stage E14, equivalent to 9 weeks in humans, the intestinal epithelium undergoes repetitive rounds of remodeling, in part instructed by the underlying mesenchyme. This leads to emergence of villus units, a process designated "villogenesis/villification" that is completed by E18.5 (Figure 1) [21]. At birth, the generated villi contain differentiated cells and the intervillus regions contain proliferating Lgr5-expressing CBC precursors that are responsive to Wnt signaling [22,23]. After birth, invagination of intervillus regions into the surrounding mesenchyme leads to formation of crypts, a niche in which reside adult stem cells together with Paneth cells [20]. Similarly, gastric glands emerge in the epithelium after a secondary specification stage taking place around E14-E15, an event also influenced by its crosstalk with the surrounding mesenchyme (Figure 1) [24].
Since 2009, efficient protocols have been developed to culture ex vivo mouse or human adult stem cells, which can be maintained in culture for long-term and spontaneously differentiate into the various cell lineages of the tissue of origin, growing as organoids [25,26]. Taking advantage of this culture system, the intestinal and gastric epithelial cells present before the onset of cytodifferentiation have been isolated and characterized (Figure 1). They grow as undifferentiated spheroids and express a transcriptome very different from the bona-fide adult Lgr5+ stem cells. Despite their dependence on autocrine Wnt ligand production for ex vivo growth, these progenitors do not rely on canonical Wnt signaling as it is reported in adult Lgr5+ cells, suggesting requirement for alternative Wnt pathways [16,27]. Despite the fact that an organ-specific commitment is already present, intestinal and gastric progenitors share many similarities at the global transcription level in vivo and ex vivo allowing to define a common progenitor signature [16,27,28]. This list involves genes associated with tissue development, cell migration/adhesion as well as cell proliferation/differentiation, processes which are obviously needed to generate brand-new villi or glands from an initial simple columnar epithelium [16]. Two prototype genes, highly expressed in these progenitors, are the cell surface molecule Trop2/Tacstd2 and the connexin Cnx43/Gja1 (Table 1). Using these markers to follow progenitor cell fate in vivo, it has been evidenced that Trop2+/Cnx43+ cells lining the E14.5 epithelium generate pre-natal intestinal villogenesis and gastric gland formation. Their minor, if any, contribution to postnatal tissue formation is explained by progressive replacement of this transient progenitor population by adult Lgr5+ stem cells emerging after the beginning of the cytodifferentiation process in intestine and stomach [16,27]. Ex vivo, fetal progenitors can be induced to mature into organoid-like structures of the tissue of origin when Wnt, Notch, gastrin and Fgf signaling pathways are modulated [16,27,29]. In vivo, a proof-of-concept experiment has demonstrated that the immature fetal intestinal cells have also the potential to mature within damaged adult colon into the appropriate regional identity, likely induced by micro-environmental cues, leading to efficient epithelial regeneration in the mouse model [29]. The signals deriving from the surrounding tissue (likely the mesenchymal compartment) that promote epithelial maturation remain to be identified. The developed alternative ex vivo culture system generating adult intestinal or gastric tissues from induced pluripotent stem cells and containing both mesenchymal and epithelial populations may help to clarify this question [30,31].
Adult Regeneration
Many efforts have been made in the past years to identify which cell types and molecular pathways are activated in adults upon injury to restore the original tissue architecture. Besides a definitive role of mesenchymal cells not reviewed here [reviews: 32,33], both reserve and facultative epithelial stem cells are involved in regeneration processes (Figure 1).
Small intestine
The actively cycling Lgr5+ stem cell pool is a radiosensitive cell type at ionizing radiation doses of 10-15 Gy. After irradiation, tissue architecture can however be maintained due to re-activation of Tert+/Bmi1+ reserve stem cells that generate new Lgr5+ cells, ensuring in this way epithelial turn-over [7,10]. A similar process occurs if Lgr5+ cells are specifically ablated with diphtheria toxin [34]. Nonetheless, if irradiation takes place in an Lgr5-depleted epithelium, regeneration is impaired providing evidence for an essential role of Lgr5+ cells to finally reconstitute tissues [35]. Interconversion between the active CBCs and the reserve stem cell pools (expressing high levels of the Hopx/Bmi1/Tert markers) has been evidenced and is, at least in part, regulated by Notch signaling [36]. In terms of gene expression, the radiation-activated reserve Sox9high stem cell pool undergoes significant transcriptomic changes favoring re-entry into the cell cycle and stimulation of DNA recombination and repair processes, but also modifications in cell adhesion/motility properties as well as shift towards downregulation of oxidative processes [4]. Noteworthy, part of this Sox9high population corresponds to terminally differentiated enteroendocrine cells that can re-acquire proliferation capacity at early post-irradiation stages and can be viewed as "facultative" stem cells [4]. Such cell plasticity induced by damage has also been reported for the Paneth secretory lineage characterized by high levels of CD24 marker expression. Indeed, upon tissue irradiation, differentiated Paneth cells upregulate Bmi1 expression while down regulating Paneth cell markers and switch back to a proliferative state [37,38]. Moreover, committed progenitors can also revert to a stem cell-like state, as demonstrated for progenitors of the secretory lineages expressing the Notch ligand Dll1+ upon irradiation [39]. Similarly, absorptive-lineage progenitors expressing Alpi down regulate enterocyte-specific markers while re-expressing the CBC markers Ascl2 and Smoc2 upon Lgr5+ stem cell loss [40]. Finally, a population of radioresistant Krt19+/Lgr5- cells, identified in the proliferative compartment above the "+4 position" zone and extending till the crypt isthmus, continue to lineage-trace upon irradiation consistent with stem cell expansion contrary to the radiosensitive CBC type [41].
Recent reports have also investigated changes in stem cell pools upon physiological alterations related to nutrition. Long-term caloric restriction sensed by Paneth cells, or high fat diet can enhance stemness of actively cycling Lgr5+ cells [42,43]. Moreover, PTEN, a negative regulator of the PI3K>AKT>mTORC1 pathway activated by growth factors like insulin and IGF1, plays a key role in maintenance of reserve stem cell pools [44]. Acute nutrient deprivation causes a transient inhibition of PTEN activity driving these cells into a "poised" state in which they are functionally ready to contribute to intestinal regeneration upon refeeding [44]. Permanent PTEN deficiency leads to exhaustion of the reserve stem cell pool that compromises efficient regeneration in case of tissue irradiation [44]. Interestingly, stimulation of reserve Sox9high cells with IGF1 enhances cell proliferation and metabolic shift towards glycolysis under radiation-induced regeneration [45]. Together, these studies point to a critical influence of the nutritional status on the efficiency of tissue regeneration.
Stomach
In the corpus, the marker Tnfrsf19/Troy labels differentiated parietal and chief cells at the gland base under steady-state conditions [14]. However, depletion of the proliferating isthmus compartment induces reactivation of differentiated Troy+ cells, which acquire stem cell-like properties and repopulate corpus glands [14].
In antral glands, hormonal treatment with progastrin increases the number of Cck2r+/Lgr5- stem cells and induces their interconversion into Lgr5-expressing cells. This activated signaling globally promotes gland fission [18]. Similarly, a rare pool of dormant Vil1-traced cells can be reactivated upon interferon gamma treatment, leading to repopulation of entire antral gland units [46]. In both cases, the potential transcriptomic changes occurring in these stem cells to promote tissue renewal remain to be investigated.
Recently, analysis of global transcripts from antral glands undergoing regeneration after depletion of the Lgr5+ stem cell pool has been reported. Soon after induction of Lgr5 ablation, differentiated cells of the mucus neck and endocrine lineages, located in proximity of the damaged area, start to express the prototype fetal progenitor marker Trop2 [16]. Over time, the majority of reactive Trop2+ cells enter the cell cycle allowing repopulation of the whole gland. As compared to adult Lgr5+ stem cells, the ones re-expressing Trop2+ show lower levels of other gastric reserve or facultative stem cell markers (Cck2r, Tnfrsf19, Vil1) [16]. Overall, re-expression of Trop2 in adult cells is associated with substantial changes in their transcriptome consistent with a shift towards a fetal-like expression pattern similar to that exhibited by fetal gastric Trop2+ or cultured spheroids, and that is characterized by activation of the whole proliferation machinery, induction of genes involved in tissue morphogenesis and organ development [16]. Another type of injury induced by high dose administration of a non steroidal anti-inflammatory drug (indomethacin) also involves re-expression of Trop2 in epithelial areas close to damaged glands, suggesting that this may represent a program commonly activated for tissue regeneration in stomach [16].
Concluding Remarks
Fundamental research in the stem cell field is instrumental to ultimately develop therapies with improved efficacy in digestive regenerative medicine. Recent studies have provided significant advances in the development of tools to isolate, culture ex vivo and analyze global transcriptome of stem cells at the single cell level. Multiple types of active or quiescent stem cells showing potential interconversion have been reported, but basic research is still needed to get a full picture of potential relationships between these various cell types and molecular pathways controlling their activated or dormant state in steady-state or regeneration conditions. Besides, in case of injury, cell dedifferentiation associated to partial and transient re-expression of a fetal program, also takes part to regeneration. Whether persistence of such regenerative process, potentially enhanced by chronic inflammation, might ultimately lead to malignant transformation, will have to be addressed in the future.
Acknowledgments
We thank Gilbert Vassart for critical reading of the manuscript. This work was supported by the Interuniversity Attraction Poles Programme- Belgian Science Policy (6/14), Belgian Fonds de la Recherche Scientifique Médicale, Walloon Region (CIBLES) and non-for-profit Association Recherche Biomédicale et Diagnostic.
References
-
Barker N, van Es JH, Kuipers J, Kujala P, van den Born M, et al. (2007) Identification of stem cells in small intestine and colon by marker gene Lgr5. Nature 449: 1003-1007.
-
Muñoz J, Stange DE, Schepers AG, van de Wetering M, Koo BK, et al. (2012) The Lgr5 intestinal stem cell signature: robust expression of proposed quiescent '+4' cell markers. EMBO J 31: 3079-3091.
-
Li N, Yousefi M, Nakauka-Ddamba A, Jain R, Tobias J, et al. (2014) Single-cell analysis of proxy reporter allele-marked epithelial cells establishes intestinal stem cell hierarchy. Stem Cell Reports 3: 876-891.
-
Van Landeghem L, Santoro MA, Krebs AE, Mah AT, Dehmer JJ, et al. (2012) Activation of two distinct Sox9-EGFP-expressing intestinal stem cell populations during crypt regeneration after irradiation. Am J Physiol Gastrointest Liver Physiol 302: G1111-1132.
-
Maria Cambuli F, Rezza A, Nadjar J, Plateroti M (2013) Brief report: musashi1-eGFP mice, a new tool for differential isolation of the intestinal stem cell populations. Stem Cells 31: 2273-2278.
-
Sangiorgi E, Capecchi MR (2008) Bmi1 is expressed in vivo in intestinal stem cells. Nat Genet 40: 915-920.
-
Montgomery RK, Carlone DL, Richmond CA, Farilla L, Kranendonk ME, et al. (2011) Mouse telomerase reverse transcriptase (mTert) expression marks slowly cycling intestinal stem cells. Proc Natl Acad Sci U S A 108: 179-184.
-
Takeda N, Jain R, LeBoeuf MR, Wang Q, Lu MM, et al. (2011) Interconversion between intestinal stem cell populations in distinct niches. Science 334: 1420-1424.
-
Powell AE, Wang Y, Li Y, Poulin EJ, Means AL, et al. (2012) The pan-ErbB negative regulator Lrig1 is an intestinal stem cell marker that functions as a tumor suppressor. Cell 149: 146-158.
-
Yan KS, Chia LA, Li X, Ootani A, Su J, et al. (2012) The intestinal stem cell markers Bmi1 and Lgr5 identify two functionally distinct populations. Proc Natl Acad Sci U S A 109: 466-471.
-
Hoffmann W (2015) Current Status on Stem Cells and Cancers of the Gastric Epithelium. Int J Mol Sci 16: 19153-19169.
-
Quante M, Marrache F, Goldenring JR, Wang TC (2010) TFF2 mRNA transcript expression marks a gland progenitor cell of the gastric oxyntic mucosa. Gastroenterology 139: 2018-2027.
-
Barker N, Huch M, Kujala P, van de Wetering M, Snippert HJ, et al. (2010) Lgr5(+ve) stem cells drive self-renewal in the stomach and build long-lived gastric units in vitro. Cell Stem Cell 6: 25-36.
-
Stange DE, Koo BK, Huch M, Sibbel G, Basak O, et al. (2013) Differentiated Troy+ chief cells act as reserve stem cells to generate all lineages of the stomach epithelium. Cell 155: 357-368.
-
Nam KT, O'Neal RL, Coffey RJ, Finke PE, Barker N, et al. (2012) Spasmolytic polypeptide-expressing metaplasia (SPEM) in the gastric oxyntic mucosa does not arise from Lgr5-expressing cells. Gut 61: 1678-1685.
-
Fernandez Vallone V, Leprovots M, Strollo S, Vasile G, Lefort A, et al. (2016) Trop2 marks transient gastric fetal epithelium and adult regenerating cells after epithelial damage. Development 143: 1452-1463.
-
Arnold K, Sarkar A, Yram MA, Polo JM, Bronson R, et al. (2011) Sox2(+) adult stem and progenitor cells are important for tissue regeneration and survival of mice. Cell Stem Cell 9: 317-329.
-
Hayakawa Y, Jin G, Wang H, Chen X, Westphalen CB, et al. (2015) CCK2R identifies and regulates gastric antral stem cell states and carcinogenesis. Gut 64: 544-553.
-
Nakajima T, Konda Y, Izumi Y, Kanai M, Hayashi N, et al. (2002) Gastrin stimulates the growth of gastric pit cell precursors by inducing its own receptors. Am J Physiol Gastrointest Liver Physiol 282: G359-366.
-
Spence JR, Lauf R, Shroyer NF (2011) Vertebrate intestinal endoderm development. Dev Dyn 240: 501-520.
-
Walton KD, Kolterud A, Czerwinski MJ, Bell MJ, Prakash A, et al. (2012) Hedgehog-responsive mesenchymal clusters direct patterning and emergence of intestinal villi. Proc Natl Acad Sci U S A 109: 15817-15822.
-
Garcia MI, Ghiani M, Lefort A, Libert F, Strollo S, et al. (2009) LGR5 deficiency deregulates Wnt signaling and leads to precocious Paneth cell differentiation in the fetal intestine. Dev Biol 331: 58-67.
-
Dehmer JJ, Garrison AP, Speck KE, Dekaney CM, Van Landeghem L, et al. (2011) Expansion of intestinal epithelial stem cells during murine development. PLoS One 6: e27070.
-
Kim TH, Shivdasani RA (2016) Stomach development, stem cells and disease. Development 143: 554-565.
-
Sato T, Vries RG, Snippert HJ, van de Wetering M, Barker N, et al. (2009) Single Lgr5 stem cells build crypt-villus structures in vitro without a mesenchymal niche. Nature 459: 262-265.
-
Sato T, Clevers H (2013) Growing self-organizing mini-guts from a single intestinal stem cell: mechanism and applications. Science 340: 1190-1194.
-
Mustata RC, Vasile G, Fernandez-Vallone V, Strollo S, Lefort A, et al. (2013) Identification of Lgr5-independent spheroid-generating progenitors of the mouse fetal intestinal epithelium. Cell Rep 5: 421-432.
-
Li X, Udager AM, Hu C, Qiao XT, Richards N, et al. (2009) Dynamic patterning at the pylorus: formation of an epithelial intestine-stomach boundary in late fetal life. Dev Dyn 238: 3205-3217.
-
Fordham RP, Yui S, Hannan NR, Soendergaard C, Madgwick A, et al. (2013) Transplantation of expanded fetal intestinal progenitors contributes to colon regeneration after injury. Cell Stem Cell 13: 734-744.
-
Finkbeiner SR, Hill DR, Altheim CH, Dedhia PH, Taylor MJ, et al. (2015) Transcriptome-wide Analysis Reveals Hallmarks of Human Intestine Development and Maturation In Vitro and In Vivo. Stem Cell Reports.
-
McCracken KW, Catá EM, Crawford CM, Sinagoga KL, Schumacher M, et al. (2014) Modelling human development and disease in pluripotent stem-cell-derived gastric organoids. Nature 516: 400-404.
-
Yen TH, Wright NA (2006) The gastrointestinal tract stem cell niche. Stem Cell Rev 2: 203-212.
-
Tarnawski AS, Ahluwalia A (2012) Molecular mechanisms of epithelial regeneration and neovascularization during healing of gastric and esophageal ulcers. Curr Med Chem 19: 16-27.
-
Tian H, Biehs B, Warming S, Leong KG, Rangell L, et al. (2011) A reserve stem cell population in small intestine renders Lgr5-positive cells dispensable. Nature 478: 255-259.
-
Metcalfe C, Kljavin NM, Ybarra R, de Sauvage FJ (2014) Lgr5+ stem cells are indispensable for radiation-induced intestinal regeneration. Cell Stem Cell 14: 149-159.
-
Srinivasan T, Than EB, Bu P, Tung KL, Chen KY, et al. (2016) Notch signalling regulates asymmetric division and inter-conversion between lgr5 and bmi1 expressing intestinal stem cells. Sci Rep 6: 26069.
-
Roth S, Franken P, Sacchetti A, Kremer A, Anderson K, et al. (2012) Paneth cells in intestinal homeostasis and tissue injury. PLoS One 7: e38965.
-
Buczacki SJ, Zecchini HI, Nicholson AM, Russell R, Vermeulen L, et al. (2013) Intestinal label-retaining cells are secretory precursors expressing Lgr5. Nature 495: 65-69.
-
van Es JH, Sato T, van de Wetering M, Lyubimova A, Nee AN, et al. (2012) Dll1+ secretory progenitor cells revert to stem cells upon crypt damage. Nat Cell Biol 14: 1099-1104.
-
Tetteh PW, Basak O, Farin HF, Wiebrands K, Kretzschmar K, et al. (2016) Replacement of Lost Lgr5-Positive Stem Cells through Plasticity of Their Enterocyte-Lineage Daughters. Cell Stem Cell 18: 203-213.
-
Asfaha S, Hayakawa Y, Muley A, Stokes S, Graham TA, et al. (2015) Krt19(+)/Lgr5(-) Cells Are Radioresistant Cancer-Initiating Stem Cells in the Colon and Intestine. Cell Stem Cell 16: 627-638.
-
Yilmaz ÖH, Katajisto P, Lamming DW, Gültekin Y, Bauer-Rowe KE, et al. (2012) mTORC1 in the Paneth cell niche couples intestinal stem-cell function to calorie intake. Nature 486: 490-495.
-
Beyaz S, Mana MD, Roper J, Kedrin D, Saadatpour A, et al. (2016) High-fat diet enhances stemness and tumorigenicity of intestinal progenitors. Nature 531: 53-58.
-
Richmond CA, Shah MS, Deary LT, Trotier DC, Thomas H, et al. (2015) Dormant Intestinal Stem Cells Are Regulated by PTEN and Nutritional Status. Cell Rep 13: 2403-2411.
-
Van Landeghem L, Santoro MA, Mah AT, Krebs AE, Dehmer JJ, et al. (2015) IGF1 stimulates crypt expansion via differential activation of 2 intestinal stem cell populations. FASEB J 29: 2828-2842.
-
Qiao XT, Ziel JW, McKimpson W, Madison BB, Todisco A, et al. (2007) Prospective identification of a multilineage progenitor in murine stomach epithelium. Gastroenterology 133: 1989-1998.
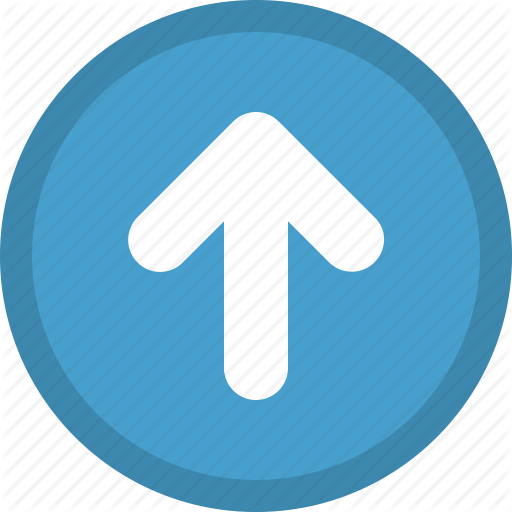