Journal of Infectious Diseases and Epidemiology
Dengue Fever: Historical Perspective and the Global Response
Tyler Warkentien* and Rebecca Pavlicek
U.S. Naval Medical Research Center- Asia, Singapore Naval Base, Singapore
*Corresponding author:
Tyler E. Warkentien, MD, U.S. Naval Medical Research Center- Asia, PSC 470 Box 4200, FPO, AP, 96534, Singapore, Tel: +65-6571-2204, E-mail: Tyler.warkentien@fe.navy.mil
J Infect Dis Epidemiol, JIDE-2-015, (Volume 2, Issue 2), Review Article; ISSN: 2474-3658
Received: May 05, 2016 | Accepted: June 29, 2016 | Published: July 02, 2016
Citation: Warkentien T, Pavlicek R (2016) Dengue Fever: Historical Perspective and the Global Response. J Infect Dis Epidemiol 2:015. 10.23937/2474-3658/1510015
Copyright: © 2016 Warkentien T, et al. This is an open-access article distributed under the terms of the Creative Commons Attribution License, which permits unrestricted use, distribution, and reproduction in any medium, provided the original author and source are credited.
Abstract
Dengue fever remains an important mosquito-borne viral illness resulting in substantial human and economic costs. Despite rising incidence rates in recent decades, there is cause for hope, particularly in light of recent advancements in dengue vaccine and vector control research. This article reviews past dengue control efforts and discusses current and future strategies for dengue control.
Keywords
Dengue fever, Dengue epidemiology, Vector control, Dengue vaccine development
Introduction
In recent years, there have been several "good news stories" in global health, such as a steady decrease in child mortality rates and malaria incidence, as well as the near elimination of polio from the planet. However, many challenges remain with regard to both emerging infectious disease outbreaks and increasing rates of some neglected tropical diseases [1]. Dengue fever is an apt example of the latter, having progressively advanced in both the number of endemic countries as well as risk for severe manifestations, such as dengue hemorrhagic fever. In the last half century, the worldwide incidence of dengue has risen 30-fold [2]. In this paper we explore the environmental and human factors that have given rise to the expansion of this disease, particularly in Asia. We also highlight the advancements already made throughout the Asia-Pacific region in combating the spread of dengue, and discuss future efforts.
Dengue fever (DF) is among the most common mosquito-borne infections in the world, yet it has long been categorized as a "neglected tropical disease [3]." Nevertheless, nearly 2.5 billion people live in dengue endemic regions, and each year an estimated 50-100 million people are infected, resulting in 500,000 hospitalizations [2,4,5] and 20,000 deaths [2]. Even these numbers are likely an under representation, since many cases are undiagnosed and unreported due to discrepancies in reporting requirements and inadequate surveillance systems. The actual annual case load may be as high as 390 million (Figure 1) [6]. Recent outbreaks in Malaysia, Taiwan, and India are among the highest those nations have faced in years [7]. Additionally, in Southeast Asia, dengue infections cost US$950 million in total and contribute 372 disability-adjusted life years per million inhabitants [8].
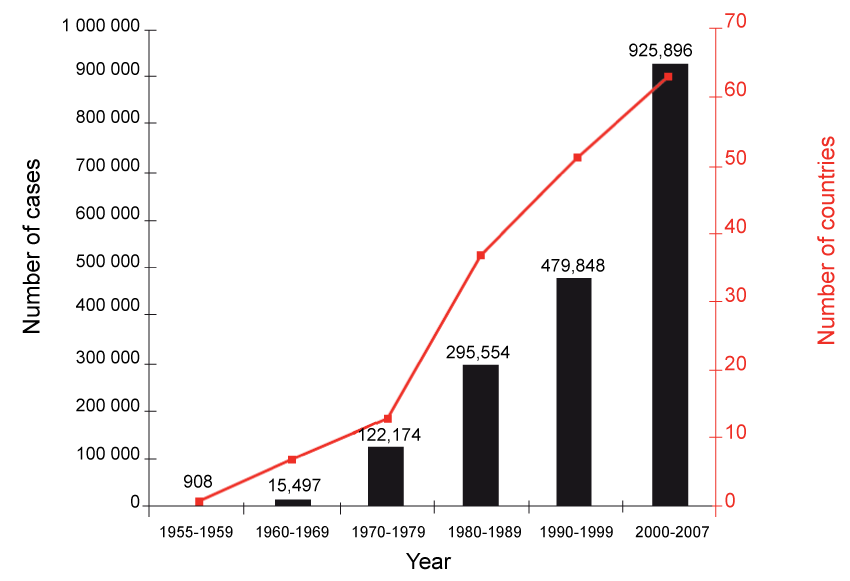
.
Figure 1: Bars indicate average annual number of dengue fever and/or DHF reported to the WHO, and line indicates total number of countries reporting cases. While these numbers have risen steadily in recent decades, they are lower than estimates of actual number of cases and countries affected for various reasons that are discussed in detail later. (From WHO, reprinted with permission) [5].
View Figure 1
Clinical and Microbiology Background
Dengue fever is caused by a flavivirus carried by an Aedes mosquito vector (Ae. aegypti and Ae. albopictus). The geographic ranges of these mosquito vectors are expanding steadily (Figure 2). Dengue fever virus does not have a broad range of animal hosts, leaving the human-mosquito cycle as essentially the sole means of transmission [9]. There are four distinct DEN serotypes (DENV1, DENV2, DENV3, and DENV 4); historically these four serotypes circulated with geographic distinction. Now, however, all four serotypes circulate in each of the global endemic regions [10]. Infection with one dengue virus serotype generally results in long term immunity to the same viral serotype. However, due to complexities of the immune response, subsequent infection with a different serotype can make an individual more susceptible to severe manifestations such as dengue hemorrhagic fever (DHF) [4]. This immunologic phenomenon partially explains why there was a rapid rise in DHF rates in the decades following World War II (WWII) as global travel steadily increased. In endemic areas with high rates of immunity to the longstanding circulating viral serotype, not only was the population generally non-immune to the newly introduced viral serotypes, but they were also "immunologically primed" to experience more severe symptoms with subsequent infections [11].
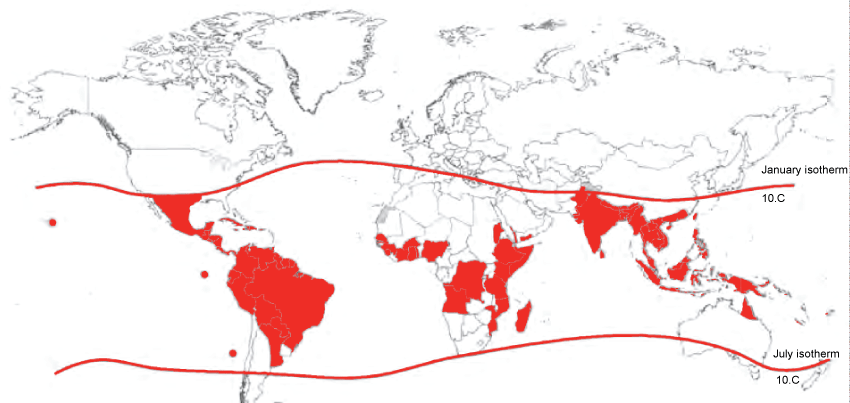
.
Figure 2: Shaded areas are countries at risk of dengue fever due to presence of Aedes mosquito, as of 2008. The contour lines are range of January/July isotherm indicating the potential range of Aedes aegypti. (From WHO, reprinted with permission) [5].
View Figure 2
The incubation period for dengue virus is five to seven days. Viremia peaks and falls sharply over the following week, thus onward transmission may occur during this time. Aedes mosquitoes have a very short average flight range, often traveling less than 500 meters' distance in their lifespan, and feed almost exclusively on humans, both indoors and out [12]. This small range requires them to live in close proximity to humans. Once an infected person becomes viremic, they may remain asymptomatic, develop a mild nondescript febrile illness, or experience a range of symptoms from fever, headache, myalgias, and rash to severe symptoms such as shock, hemorrhage and organ failure. Severe dengue fever arises primarily due to vascular permeability; the immune response to the virus causes fluid to leak out of the vasculature into tissue spaces, causing shock, organ failure, and hemorrhage [4,5]. Host immune factors are likely primarily responsible for initiating the vascular permeability and plasma loss that characterize dengue infection [4].
The 1997 World Health Organization (WHO) classification scheme [13] categorized the disease as dengue fever, DHF, or dengue shock syndrome (DSS). Unfortunately, this classification system had shortcomings. Many of the criteria used as markers of severity required clinical judgment and led to inconsistencies in the epidemiology and classification [14,15]. There were also four different levels of severity for DHF [13], adding even more complexity to the classification. In 2009 the WHO established new diagnosis and management guidelines, altering the case definitions [5]. There are now three categories: DF, DF "with warning signs" and severe dengue (SD). As such, these prognostic criteria provided clinicians with a more measurable way of identifying those who are likely to develop SD, and may require more intensive monitoring and support earlier in the disease course [5]. Even with the 2009 WHO diagnostic criteria, shortcomings remain, as altered hemostasis is not factored [16]. At this time there is no reliable prognostic laboratory test to predict the development of SD. Clinical studies suggest an association between cytokines or mast-cell derived mediators such as chymase, vascular endothelial cell growth factor and IL-10 and severity [17-19]. Importantly, a mechanism was recently shown by which dengue NS1 protein disrupts endothelial cellular integrity [20,21], which could contribute to disease severity.
Historical Picture
Prior to WWII, dengue fever occurred in coastal cities throughout the tropics and subtropics, with epidemics occurring periodically. Ae. aegypti is thought to have originated in Africa, while Ae. albopictus likely originated in Asia. Nevertheless, during the age of exploration and rise of international trade during the 17th and 18th centuries, dengue fever spread to tropical cities worldwide, likely due to expansion of Ae aegypti from Africa to Asia and the Americas [22]. Epidemics of dengue were reported in 1635 in the Caribbean and 1699 in Central America [10]. The name "dengue" derives from a word meaning "affected" used in the Caribbean to describe these outbreaks. The history of dengue fever is complicated by the fact that some outbreaks in the Caribbean during the nineteenth century may have been chikungunya, an alphavirus presenting with clinical manifestations that were called "dengue" at the time [23]. Once the rise of coastal urban centers and international shipping occurred, dengue became more pervasive and more easily recognized. Since urban centers in the tropics were relatively small, and transportation between them was generally by ocean going ships, epidemics were minimized and each region had only one or two circulating virus strains, so dengue carried little public health importance [11].
During WWII, due to massive movement of troops, particularly into remote regions of Southeast Asia and the Pacific Islands, dengue spread dramatically. Dengue had a major impact on troop strength in the Pacific during WWII, and along with malaria, was one of the two major disease threats. It has remained an important infectious threat to the US military, as it has accounted for 20-30% of undifferentiated fever in Vietnam, Somalia, and Haiti [24]. Its impact on the region was greater still. By the end of WWII, as a result of the vector and the virus having been spread throughout Asia-Pacific nations, many Asian countries were hyperendemic with all four viruses [11]. Following WWII, sustained economic growth in many Asian cities led to rapid population growth. With this spread of globalization and urbanization, particularly in Southeast Asia, the stage was set for dengue hemorrhagic fever epidemics. The first such epidemic was reported in the Philippines (1953-54), followed by Thailand (1958), then Malaysia, Singapore, Vietnam, Indonesia, and Myanmar (Burma) in the 1960s and 1970s. By the 1980s DHF was a leading cause of childhood illness and death in some Southeast Asian nations [11].
Latin America experienced similar population growth and urbanization in the post-WWII era. DHF epidemics in the Americas were curtailed though, through a major public health campaign. During the decades following WWII, a broad scale international campaign by the Pan American Health Organization (PAHO) sought to eliminate the Ae. aegypti vector from the hemisphere. While yellow fever elimination was the target of the campaign, dengue rates would decline as well since the viruses share vectors. This effort began with major backing from government officials, significant funding mechanisms, and widespread public health engagement. The mosquito was eliminated from the majority of Brazil, and similar successes were seen in other South American nations. By 1947, with the widespread use of DDT insecticide, hopes were high and a continental Ae. aegypti eradication plan was introduced. Despite such broad scale efforts, the early successes were not sustained. Dengue outbreaks occurred during the 1960s and an Asian dengue virus, DEN3, was reintroduced to the Caribbean [25]. PAHO remained committed to the eradication effort, but political will, funding, coordination, and surveillance decreased, while globalization, international travel, and urbanization were on the rise. Unfortunately, as the broad coalition of support for this noble effort waned, Ae. aegypti returned, and eventually surpassed, its prior geographic range. Not surprisingly, dengue rates rose steadily throughout the Americas in the 1970s and 1980s, reaching pre-campaign levels by 1995 [11].
The spread of dengue can be closely tied to both the geographic spread, as well as the domestication of its vectors. The primary vector, Ae. aegypti is anthropophagic (preference for human blood-meals), and when species evolve the ability to co-exist with humans, they often spread by human mobility [22]. Ae. aegypti likely originated in Africa, then arrived in the Americas with the first Europeans, with domestication occurring either before or simultaneous with its arrival in the New World [22]. Regarding Asian Ae aegypti, historical data suggests African origin as well, whereas genetic data points to subsequent spread from the Americas. Nevertheless, the present day worldwide domestic Ae. aegypti is a monophyletic group, suggesting a point origin for domestication, and all populations outside of Africa arose from this lineage [22]. The expansion of dengue fever can be tied to the ecology of Ae. albopictus and its spread from its geographic origin as well. It was first recorded in Europe in 1979, and in the continental US in 1985 [26]. The limited phylogeographic difference in genetics provides evidence for expansion due to human activities [26].
Current Landscape
While all major regions of the world are affected by increasing dengue rates, nearly 75% of those exposed to dengue live in the Asia-Pacific region [27], and rates of DHF in southeast Asia are 18 times higher than in the Americas [10]. Cases have continued to increase over the last decade in Cambodia, Laos, Malaysia, Singapore, Philippines and Vietnam. Dengue's impact on lost productivity is also notable. The DALY burden of dengue is estimated to be 700,000 per year worldwide, primarily affecting children [10]. The economic burden is difficult to assess, but studies suggest an annual cost of $2.1 billion in the Americas and $950 million in SE Asia. These studies notably exclude the cost of prevention [10]. Providing an estimate of dengue's actual impact is difficult as there are extensive variations in national reporting data, and many nations don't have a robust reporting system [28]. As a result, in 2010 there were 2.2 million cases reported to the WHO, but an estimate of 96 million actual cases [6]. In India, where there is no mandatory centralized reporting, there are estimated to be 282 dengue infections for every reported case [29].
Even if the case load estimate was more accurate, determining the economic burden is even more difficult. Additionally, some economic impact areas, such as impact on tourism, have not been fully explored [30]. Most commonly, disease burden is calculated using the acute illness of seven days or less standard. However, there are increasing reports of long term post-viral effects, such as chronic fatigue syndrome and depression [30]. Ideally, the overall impact of the impaired quality of life and poverty rates should somehow be factored into disease burden estimates. Due to domestication and anthropophagy (predilection for human feeding vice other vertebrates) of the Aedes vectors, dengue is a predominantly urban disease. With rapid population growth in many urban centers, the urban poor are predominantly affected by dengue. These population groups have limited access to domicile amenities that may prevent indoor mosquito biting such as air conditioning and screening of windows and doors.
The expanding geographic niche of Aedes is due in part to adaptation by the mosquito. While the Ae. aegypti has long been found in urban environments, Ae. albopictus has historically been known as a sylvatic species, often remote from large human populations. However, the mosquito has recently undergone domestication, adapting its breeding sites from forest tree-holes to domestic and industrial man-made containers [31]. A major driver for the expansion of this vector, including its reintroduction into Europe, is the international trade of used tires, which are used as breeding sites [32]. It can tolerate sub-freezing temperatures and hibernate in small habitats, allowing it to survive the journeys involved in international shipping and develop endemicity in the countries of Europe and North America [2].
In areas where A. aegypti is non-endemic, A. albopictus is the primary vector of dengue. It is endemic in Southeast Asia, and by the 1990s it had spread globally. It has recently been implicated as the cause of either dengue or chikungunya outbreaks in Hawaii, Central Africa, and China, and was the source of the first autochthanous transmission in Europe [26]. While Ae. albopictus is a less competent vector than Ae. aegypti, high mosquito density has been shown to contribute to several arbovirus outbreaks due to Ae. Albopictus [26].
Towards a Global Response
In an effort to lead a coordinated response, the WHO launched a global strategy in 2012, with the primary goal being to reduce dengue-related mortality by 50% and morbidity by 25% by 2020 [2]. The intent is to refocuson preventive measures, risk assessments, and early warning systems, guided by research input. The keys to reducing mortality include early case detection and appropriate identification and management of severe cases. Investments in healthcare infrastructure, capacity building, improved education, and advancing research will all aid in the global dengue response. However, such efforts will also need to be coordinated by strong leadership by the WHO, the affected regions, and ministries of health in endemic countries. Thus, the WHO global strategy 2012-2020 points out the importance of measuring the cost of implementing the strategy at the national and regional levels. In addition to the stated mortality and morbidity goals, the plan called for estimating the true burden of dengue disease. To achieve these goals, the WHO puts forth five technical elements: diagnosis and case management, integrated surveillance and outbreak preparedness, sustainable vector control, future vaccine implementation, and basic operational research [2].
The first element is improving diagnosis and case management. The key to preventing severe dengue is early recognition, which allows for appropriate intravenous rehydration to counteract the plasma leakage. Therefore, if patients can be accurately diagnosed and triaged early on, mortality from dengue can be reduced to almost zero [2]. Reliance on only clinical symptoms to make the diagnosis is common practice, but leads to diagnostic errors since many other febrile diseases present similarly. Some diagnostic laboratory tools, such as molecular diagnosis (RNA polymerase chain reaction) and viral culture are available in tertiary care centers and reference labs, but often not available in real time to clinicians in the field. The detection of the NS1 viral antigen can be detected early during the febrile period though, and there are now several commercial products available with a sensitivity ranging from 72% to 89% [33]. IgM antibody tests, which are more useful later in the disease course, are becoming available as a rapid test also.
Improvements in surveillance systems are necessary for early outbreak detection, to better estimate the true burden of disease, and to evaluate the effectiveness of dengue control programs. Since collecting complete country level data is difficult in most dengue endemic nations, the establishment of sentinel sites for surveillance should be pursued [2]. On an international level, the WHO has an established Global Outbreak Alert and Response Network that can respond rapidly to a developing outbreak when summoned. This early and swift effort could prevent an outbreak before one occurs. Data from outbreaks is often dependent on inaccurate reporting systems. National case reporting mechanisms could be improved by using expansion factors, which adjust for underreporting using available empiric data. Quantitative modeling could also help to achieve a more accurate measurement, taking into account the many factors on dengue transmission, such as climate, season, urbanization and mosquito population [28].
Epidemiologic surveillance is important, but efforts must be complemented by integrated vector management (IVM). This leads to the third arm of the WHO plan, namely sustainable vector control. Mosquito vectors lead to other infections such as malaria and chikungunya, so an IVM approach provides control for multiple pathogens. The goal is to eliminate mosquito breeding sites ranging from small open domestic containers to industrial water collection systems. Many dengue endemic nations have public health campaigns to alert the public of such breeding sites, such as used tires and open top water containers. Singapore conducts a national pupal collection survey to identify the items around the house that are the most proficient breeding sites such as flower pots and ornamental containers [34]. Indoor residual spraying is another vector control tool that is commonly used in malaria control efforts, but Aedes, unlike the Anopheles vector of malaria, does not rest very long on walls and other indoor surfaces, making this technique less effective for dengue control [2]. Another challenge with vector control is the potential of Aedes to develop resistance to the insecticides. This is one factor that led to the eventual failure of the PAHO Aedes elimination program [25]. Resistance among Ae. Aegypti to organophosphates and pyrethroids is already widespread [2].
Despite these challenges, new and innovative methods of vector control are being deployed. As part of the Eliminate Dengue program, an Australian-based research team is harnessing the properties of a bacteria that exists as a naturally occurring symbiont of many insect species [12]. Wolbachia is an obligate intracellular bacterium that exists in cytoplasm of cells within many insect species naturally. It is typically transmitted vertically through the maternal cytoplasm and is a non-pathogen in humans [35]. Wolbachia does not occur naturally in the Ae aegypti mosquito, but when inserted by transgenics, it reduces the ability of the mosquito to transmit the dengue virus [12]. This occurs in multiple ways. First, Wolbachia exhibits a reproductive manipulation technique called cytoplasmic incompatibility (CI). When Wolbachia-infected males mate with either a Wolbachia-naïve female or a female with an incompatible Wolbachia, the offspring become non-viable through unidirectional or bidirectional CI, respectively [35,36]. One public health approach is to release Ae aegypti males that are artificially infected with Wolbachia into the environment, with hypothesis that CI principle will drive mosquito population down [12].
While initially developed to harness the CI principle in vector control, it was discovered that certain strains of Wolbachia reduce susceptibility to pathogens, including RNA viruses such as dengue. While the molecular basis for this phenotype that can effect pathogenesis is unknown [36], these transinfected strains known as wMelPop, wMel, and wAlbB can reduce the viral load and transmission of dengue [35]. Additionally, when females are infected with a particular strain of Wolbachia the mosquito's lifespan has been shown to decrease by 50% [37]. Research efforts have now extended into dengue endemic countries. Brazil, which has more dengue cases than any other country in the world [2], released the first Wolbachia mosquitoes in September 2014 in Rio de Janeiro [12]. In Indonesia, the Eliminate Dengue Program in partnership with Indonesian authorities began releasing Wolbachia mosquitoes around Yogyakarta, East Java, in early 2014 [12]. After four months, 66-78% of mosquitoes were Wolbachia infected, so an effect on dengue rates seems promising.
In addition to Wolbachia infected mosquitoes, there are a number of promising transgenic mosquito strains that have shown promise as a possible method to combat mosquito borne illnesses. In addition to the sterile insect technique (SIT) using Wolbachia, other SIT methods such as irradiation have been tried in effort to limit mosquito populations. While problems have limited the broad scale implementation of irradiation, a recent advancement in transgenics shows promise. In RIDL, or Release of Insects carrying a Dominant Lethal, a dominant lethal gene is inserted, but its expression is initially repressed. This feature gives this method an advantage over irradiation and CI which kill affected embryos, while RIDL affects later stages of development [36].
In addition to vector control strategies, the development of a dengue vaccine is critical, as containment of dengue will be unlikely to occur by vector and outbreak control measures alone [38]. For instance, despite a high level of focus on preventive measures and surveillance, even Singapore has seen rising rates, finding that the pervasive nature of the urban-dwelling vector is extremely difficult to control. With globalization bound to expand in the decades ahead, even if a particular city or country is able to effectively limit dengue virus and its vectors, its gains are likely to be temporary in the absence of an effective vaccine. An effective dengue vaccine could provide widespread population immunity even if Aedesis re-introduced through global travel, trade, or other means. The main challenge to vaccine development has been that a dengue vaccine must be quadrivalent, inducing effective immunity against all four dengue serotypes. The reasons behind this is the theoretical risk of antibody mediated enhancement, which would have the potential of generating more severe dengue in individuals who gain only partial immunity through vaccination [4]. The long standing concern of dengue vaccine development is that a vaccine that does not neutralize quadrivalently, could potentially induce antibody dependent enhancement in a vaccinated individual who is later exposed to dengue naturally.
Despite challenges, dengue vaccine research is an area of recent success. The most advanced vaccine is a live-attenuated chimeric yellow-fever dengue virus vaccine (CYD-TDV, Sanofi-Pasteur) [38-40]. Yellow fever is a closely related Flavivirus, and the yellow fever vaccine is a highly successful vaccine given to millions of people since the 1950s, and its properties provide a backbone for a chimeric dengue vaccine. In the first study, 6,851 children in five Asian countries received the vaccine in a three immunization series. The overall vaccine efficacy was 56%. This response was more robust in older children than younger, and also better in those with pre-existing partial immunity than those with no prior immunity. Efficacy against serotypes 3 and 4 were 65% and 72% respectively, while serotype 2 exhibited only a 35% response rate. Most importantly, efficacy against DHF was 80%, and fewer dengue patients in the vaccine group were hospitalized compared to the control group. There also was no increase in serious adverse events in the vaccine group [39]. The second study, conducted in five Latin American nations, had similar results. This study resulted in a vaccine efficacy of 60% overall, a 95% efficacy against severe dengue, and 80% against dengue hospitalization [40]. These results are encouraging, particularly with such a robust response in preventing severe disease. Also encouraging is the absence of antibody dependent enhancement toward severe disease.
Several other vaccines are in earlier stages of development and show promise as well. A purified inactivated tetravalent vaccine (TDENV PIV, GlaxoSmithKline) developed by the Walter Reed Army Institute of Research has recently been shown to induce long-lasting antibody response in an animal model dengue challenge [41], and a monovalent version (DENV-1 PIV) was safe and immunogenic in a phase I study [42]. A recombinant live attenuated tetravalent dengue vaccine (DENVax, Takeda Pharmaceuticals) was safe and immunogenic in phase I testing of flavivirus-naïve adults as well [43]. Promising results have also been shown by vaccines developed by the US National Institute of Health. Two live attenuated tetravalent vaccines (TV003, and TV005 which has an enhanced DENV-2 component) were studied in a randomized placebo-controlled trial of flavivirus-naïve patients. TV005 showed a remarkable tetravalent response in 90% of vaccinees by three months after a single dose [44]. The potential for a protective immune response with a single dose vaccine holds great public health potential, particularly for populations unprimed by previous dengue exposure such as children and travelers [45]. Despite advancements in vaccine development, issues with vaccine efficacy remain, and there are also concerns regarding vaccine delivery. Now that a licensed dengue fever vaccine (CYD-TDV) exists, endemic countries must decide who to vaccinate, assuming limited resources and likely high costs. How to best expand any dengue vaccine into existing national vaccine delivery systems must be explored. Finally, a dengue vaccination program must be well integrated into other ongoing dengue control efforts on a national and regional level [2].
The final arm of the WHO strategy is to improve basic and operational research. Some of these areas of research need are in clinical care, while other needed research tools are in public health. In addition to these focus areas, the WHO has also identified five enabling factors that will be necessary to achieve these goals: global advocacy, coordination/collaboration, communication, capacity-building, and monitoring/evaluation [2].To achieve necessary research funding, advocacy for dengue awareness must improve. Dengue awareness is beginning to grow. For instance, the Association of South East Asian Nations has designated June 15 to be "Dengue Day [30]." Each year a regional meeting is held to raise awareness and mobilize resources. Also, dengue awareness may be increased through Brazil's hosting of the 2014 World Cup and the 2016 summer Olympics as well [46,47].
In order to control dengue, a coordinated, sustained response is essential. The Pan-American efforts that were so successful during the 1950s eventually crumbled when funding and collaborations dissolved. While collaboration is necessary on a regional level, it is important to develop a coordinated response on a local level too. In Singapore, the National Environment Agency (NEA) includes sophisticated methods to collect data and report to the community [34]. The NEA website has nearly real-time geospatial updates of case detection, and a team of volunteers increases awareness on social media and reports new cases, thus serving effectively as both a public health message system and an early warning system. While this highly integrated system was implemented in 2013, it remains too early to tell its impact of dengue incidence rates. Community-based, "bottom up" communication for behavioral impact (COMBI), as promoted by WHO [2,27] is a relatively new approach in dengue control. COMBI incorporates lessons learned into public health messaging, and involving community members in the messaging is critical to its success. One example of COMBI success is in Thailand, where there is one village health worker for every ten households. They communicate dengue awareness to the community, and warn about disease outbreaks [27]. In other instances the COMBI approach has been less successful as funding and resources decreased [48].
Historically dengue research has been under-funded [2], but funding efforts have shown recent signs of improvement. The European Commission launched the "Comprehensive control of Dengue fever under changing climatic condition," providing 18 million Euros to three consortia to conduct dengue related research [49]. The US National Institute of Allergy and Infectious Diseases funds 60 dengue fever projects, including work on a vaccine [50], and the development of a tetravalent dengue vaccine remains a priority of US military research efforts as well [51]. The Bill and Melinda Gates Foundation identifies dengue fever as a "high opportunity target," funding research to improve vaccine delivery and early outbreak detection [52].
Conclusion
Dengue fever has been an important cause of human disease for centuries, with incidence rates climbing in recent decades. Analysis into why rates have risen highlights the importance of human factors such as globalization, travel, urbanization, and climate change. This review brings to light the importance of political will, public health communication, and international coordination into dengue fever control. Although efforts have fallen short in the past, there is hope for the future. Innovative vector control techniques are now being studied, and the first phase III trials of a dengue vaccine have shown efficacy on two continents, particularly showing reductions in dengue hospitalizations. If the advancing science of dengue control can be coupled with improved funding and international coordination, then the tide can be turned. Most importantly if such gains can be sustained through political will and persistent public health communication and surveillance, then the scourge of dengue fever could one day take its place alongside other infections as being primarily a disease of the past.
Disclaimer
Disclaimer for the authors who are U.S. military service members, or U.S. government employees. This work was prepared as part of their official duties. Title 17 U.S.C. §105 provides that ‘Copyright protection under this title is not available for any work of the United States Government.' Title17 U.S.C. §101 defines a U.S. Government work as work prepared by military service members or employees of the U.S. Government as part of that person's official duties. The views expressed in this article are those of the authors and do not necessarily reflect the official policy or position of the Department of the Navy, Department of Defense, nor the U.S. Government.
References
-
Bill Gates (2014) Speech to American Society of Tropical Medicine and Hygiene.
-
World Health Organization (2012) Global Strategy for Dengue Prevention and Control, 2012-2020. Geneva: WHO Press.
-
Hotez PJ, Fenwick A, Savioli L, Molyneux DH (2009) Rescuing the bottom billion through control of neglected tropical diseases. Lancet 373: 1570-1575.
-
Halstead SB (2007) Dengue. Lancet 370: 1644-1652.
-
World Health Organization (2009) Dengue: Guidelines for Diagnosis, Treatment, Prevention and Control.
-
Bhatt S, Gething PW, Brady OJ, Messina JP, Farlow AW, et al. (2013) The global distribution and burden of dengue. Nature 496: 504-507.
-
Woodall JP, Yuill TM (2016) Why is the yellow fever outbreak in Angola a ‘threat to the entire world'? International Society for Infectious Diseases.
-
Shepard DS, Undurraga EA, Halasa YA (2013) Economic and disease burden of dengue in Southeast Asia. PLoS Negl Trop Dis 7: e2055.
-
Mandell G, Bennett JE, Dolin R (2009) Mandell, Douglas, and Bennett's Principles and Practice of Infectious Diseases. (7th edn), Churchill Livingstone.
-
Murray NE, Quam MB, Wilder-Smith A (2013) Epidemiology of dengue: past, present and future prospects. Clin Epidemiol 5: 299-309.
-
Gubler DJ (2011) Dengue, Urbanization and Globalization: The Unholy Trinity of the 21st Century. Trop Med Health 39: 3-11.
-
Eliminate Dengue Program.
-
World Health Organization (WHO) (1997) Dengue Haemorrhagic Fever: Diagnosis, Treatment, Prevention and Control.
-
Bandyopadhyay S, Lum LC, Kroeger A (2006) Classifying dengue: a review of the difficulties in using the WHO case classification for dengue haemorrhagic fever. Trop Med Int Health 11: 1238-1255.
-
Horstick O, Jaenisch T, Martinez E, Kroeger A, See LL, et al. (2014) Comparing the Usefulness of the 1997 and 2009 WHO Dengue Case Classification: A systematic Literature Review. Am J Trop Med Hyg 91: 621-634.
-
Halstead SB, Cohen SN (2015) Dengue Hemorrhagic Fever at 60 years: Early Evolution of Concepts of Causation and Treatment. Microbiol Mol Biol Rev 79: 281-291.
-
St John AL, Rathore AP, Raghavan B, Ng ML, Abraham SN (2013) Contributions of mast cells and vasoactive products, leukotrienes and chymase, to dengue virus-induced vascular leakage. Elife 2: e00481.
-
Brasier AR, Ju H, Garcia J, Spratt HM, Victor SS, et al. (2012) A Three- Component Biomarker Panel for Prediction of Dengue Hemorrhagic Fever. Am J Trop Med Hyg 86: 341-348.
-
Furuta T, Murao LA, Lan NTP, Huy NT, Huong VTQ, et al. (2012) Association of Mast Cell-Derived VEGF and Proteases in Dengue Shock Syndrome. PLoS Negl Trop Dis 6: e1505.
-
Modhiran N, Watterson D, Muller DA, Panetta AK, Sester DP, et al. (2015) Dengue virus NS1 protein activates cells via Toll-like receptor 4 and disrupts endothelial cell monolayer integrity. Sci Transl Med 7: 304ra142.
-
Beatty PR, Puerta-Guardo H, Killingbeck SS, Glasner DR, Hopkins K, et al. (2015) Dengue virus NS1 triggers endothelial permeability and vascular leak that is prevented by NS1 vaccination. Sci Transl Med 7: 304-311.
-
Powell JR and Tabachnick WJ (2013) History of domestication and spread of Aedes aegypti--a review. Mem Inst Oswaldo Cruz 108: 11-17.
-
Halstead S (2015) Reappearance of Chikungunya, formerly called dengue, in the Americas. Emerg Infect Dis 21: 557-561.
-
Walter Reed Army Institute of Research.
-
Dick OB, San Martin JL, Montoya RH, del Diego J, Zambrano B, et al. (2012) The History of Dengue Outbreaks in the Americas. Am J Trop Med Hyg 87: 584-593.
-
Bonizzoni M, Gasperi G, Chen X, James AA (2013) The invasive mosquito species Aedes albopictus: current knowledge and future perspectives. Trends Parasitol 29: 460-468.
-
WHO Regional Office for South-East Asia (2011) Comprehensive Guidelines for Prevention and Control of Dengue and Dengue Haemorrhagic Fever. Revised and expanded edition.
-
Shepard DS, Undurraga EA, Betancourt-Cravioto M, Guzmán MG, Halstead SB, et al. (2014) Approaches to Refining Estimates of Global Burden and Economics of Dengue. PLoS Negl Trop Dis 8: e3306.
-
Tyagi BK, Karthiga S, Vidya C, Arora NK, Nandan D, et al. (2014) Estimation of the adjustment factor for hospitalized clinically cases diagnosed and tested for dengue in Madurai Tamil Nadu (India). Dengue Bull.
-
World Health Organization (2013) Marking ASEAN Dengue Day.
-
Beebe NW, Ambrose L, Hill L, Davis JB, Hapgood G, et al. (2013) Tracing the Tiger: Population genetics provides valuable insights into the Aedes (Stegomyia) albopictus invasion of the Australasian Region. PLoS Negl Trop Dis 7: e2361.
-
Reiter P, Sprenger D (1987) The used tire trade: a mechanism for the worldwide dispersal of container breeding mosquitoes. J Am Mosq Control Assoc 3: 494-501.
-
Lima Mda R, Nogueira RM, Schatzmayr HG, dos Santos FB (2010) Comparison of Three Commercially Available Dengue NS1 Antigen Capture Assays for Acute Diagnosis of Dengue in Brazil. PLoS Negl Trop Dis 4: e738.
-
(2016) National Environment Agency, Singapore.
-
Johnson KN (2015) The Impact of Wolbachia on Virus Infection in Mosquitoes. Viruses 7: 5705-5717.
-
Alphey L, McKemey A, Nimmo D, Oviedo MN, Lacroix R, et al. (2013) Genetic Control of Aedes mosquitoes. Pathog Glob Health 107: 170-179.
-
McGraw EA, O'Neill SL (2013) Beyond insecticides: new thinking on an ancient problem. Nat Rev Microbiol 11: 181-193.
-
Thomas SJ (2015) Preventing Dengue - Is the Possibility Now a Reality? N Engl J Med 372: 172-173.
-
Capeding MR, Tran NH, Hadinegoro SR, Ismail HI, Chotpitayasunondh T, et al. (2014) Clinical efficacy and safety of a novel tetravalent dengue vaccine in healthy children in Asia: a phase 3, randomised, observer-masked, placebo-controlled trial. Lancet 384: 1358-1365.
-
Villar L, Dayan GH, Arredondo-García JL, Rivera DM, Cunha R, et al. (2014) Efficacy of a Tetravalent Dengue Vaccine in Children in Latin America. N Engl J Med 372: 113-123.
-
Fernandez S, Thomas SJ, De La Barrera R, Im-Erbsin R, Jarman RG, et al. (2014) An adjuvanted, tetravalent dengue virus purified inactivated vaccine candidate induces long-lasting and protective antibody responses against dengue challenge in rhesus macaques. Am J Trop Med Hyg 92: 698-708.
-
Martinez LJ, Lin L, Blaylock JM, Lyons AG, Bauer KM, et al. (2015) Safety and Immunogenicity of a Dengue Virus Serotype-1 Purified-Inactivated Vaccine: Results of a Phase I Clinical Trial. Am J Trop Med Hyg 93: 454-460.
-
Osorio JE, Velez ID, Thomson C, Lopez L, Jimenez A, et al. (2014) Safety and immunogenicity of a recombinant live attenuated tetravalent dengue vaccine (DENVax) in flavivirus-naïve healthy adults in Columbia: a randomized, placebo-controlled, phase 1 study. Lancet Infect Dis 14: 830-838.
-
Kirkpatrick BD, Durbin AP, Pierce KK, Carmolli MP, Tibery CM, et al. (2015) Robust and Balanced Immune Response to All 4 Dengue virus Serotypes following Administration of a single dose of a Live Attenuated Tetravalent Dengue Vaccine to Healthy, Flavivirus-Naïve Adults. J Infect Dis 212: 702-710.
-
McArthur MA, Edelman R (2015) A Promising, Single-Dose, Live Attenuated Tetravalent Dengue Vaccine Candidate. J Infect Dis 212: 681-683.
-
Wilson ME, Chen LH, Han PV, Keystone JS, Cramer JP, et al. (2014) Illness in travelers returned from Brazil: The GeoSentinel experience and implications for the 2014 FIFA World Cup and the 2016 Summer Olympics. Clin Infect Dis 58: 1347-1356.
-
(2014) Brazil 2014: World Cup dengue fever risk predicted. BBC News Health report.
-
Azmawati MN, Aniza I, Ali M (2013) Evaluation of communication for behavioral impact (COMBI) program in dengue prevention: A qualitative and quantitative study in Selangor, Malaysia. Iran J Public Health 42: 538-539.
-
Jaenisch T, IDAMS, Sakuntabhai, Denfree, Wilder-Smith A, et al. (2013) Dengue research funded by the European Commission scientific strategies of three European dengue research consortia. PLoS Neg Trop Dis 7: e2320.
-
(2016) Dengue fever. National Institute of Allergy and Infectious Disease.
-
Military Infectious Disease Research Program.
-
` Neglected Infectious Diseases Strategy Overview.
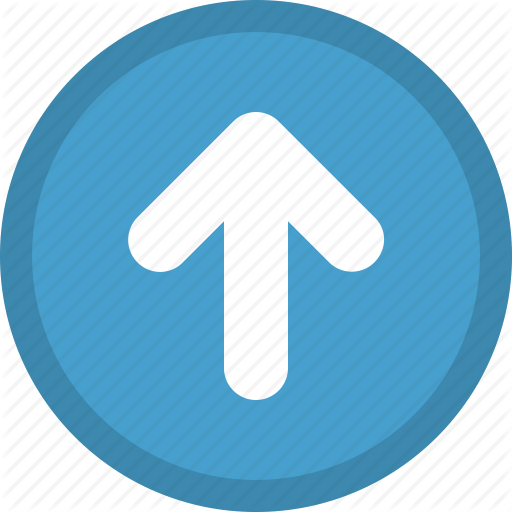