International Journal of Diabetes and Clinical Research
Recent Advances in Insulin Therapy for Diabetes
Aiman Ahmad, Iekhsan Othman, Anuar Zaini Md Zain and Ezharul Hoque Chowdhury*
Jeffrey Cheah School of Medicine and Health Sciences, Monash University, Malaysia
*Corresponding author:
Ezharul Hoque Chowdhury, Jeffrey Cheah School of Medicine and Health Sciences, Monash University, Malaysia, Tel: +603-5514-4978; Fax: +603-5514-6323; E-mail: md.ezharul.hoque@monash.edu
Int J Diabetes Clin Res, IJDCR-1-006, (Volume 1, Issue 1), Review Article; ISSN: 2377-3634
Received: October 01, 2014 | Accepted: October 23, 2014 | Published: October 27, 2014
Citation: Ahmad A, Othman I, Md Zain AZ, Chowdhury EH (2014) Recent Advances in Insulin Therapy for Diabetes. Int J Diabetes Clin Res 1:006. 10.23937/2377-3634/1410006
Copyright: ©2014 Ahmad A, et al. This is an open-access article distributed under the terms of the Creative Commons Attribution License, which permits unrestricted use, distribution, and reproduction in any medium, provided the original author and source are credited.
Abstract
Diabetes mellitus is a chronic disease affecting a great number of the world's population. Insulin therapy for diabetes is most commonly delivered via subcutaneous injections, up to four times a day. Long-term insulin therapy, compounded by the invasive nature of its administration, has caused problems with patient compliance, ultimately influencing patient outcomes. Extensive research has been carried out to date, to explore possible improvements to insulin therapy for diabetic patients, with some new products already available and FDA-approved. This review aims to highlight the new discoveries in novel insulin formulations, such as novel injectable insulins, artificial pancreas systems, oral insulins, transmucosal insulins including buccal insulins, pulmonary/inhaled insulins, nasal insulins, ocular insulins, and rectal insulins, as well as transdermal insulins, from preliminary research data to market available insulin products, and finally discuss potential future directions of insulin therapy.
Keywords
Diabetes, Insulin, Insulin delivery, Injectable insulin, Oral insulin, Transmucosal insulin, Buccal insulin, Pulmonary insulin, Inhaled insulin, Nasal insulin, Ocular insulin, Rectal insulin, Transdermal insulin
Introduction
Diabetes mellitus (DM) is a chronic disease that is caused by defective pancreatic insulin production - type 1 DM (T1DM, previously known as insulin-dependent or juvenile-onset DM), or insulin resistance - type 2 DM (T2DM, previously known as non-insulin-dependent or adult-onset DM). The disease results in hyperglycaemia which can lead to multi-organ damage in the long run, e.g. vasculopathy, neuropathy, etc. DM causes significant rates of morbidity and mortality to the current population [1].
Insulin is used to control the level of blood glucose in patients with DM. It is an essential therapy for patients suffering from T1DM, and T2DM (especially in late-stage disease).Insulin was discovered in 1921 by Frederick Banting and Charles Best, went through its first clinical use in 1922 [2-4], and helped revolutionise the treatment of T1DM which was fatal at that time. Initially, insulin was isolated from bovine and porcine pancreata, until the 1980s. Later on, recombinant DNA techniques allowed the manufacture of human insulin. Modifications of the amino acid sequence of the insulin molecule by rDNA and protein engineering methods have recently allowed the production of monomeric insulin analogues (e.g. lispro, aspart) which have a more rapid absorption profile [3].
Since DM results in a defect in insulin function, the ideal treatment is to allow diabetics to regain normal insulin function. However, current research and technology has not been able to achieve this. The current insulin treatment involves exogenous administration, with the aim of achieving effective glycaemic control (i.e. prevention of hyper- and hypoglycaemia) and avoidance of the complications of DM [5]. Current modes of delivering insulin include intravenous (IV) infusion and subcutaneous (SC) injections. SC insulin preparations, which are more commonly used, include rapid-, intermediate- and long-acting insulin, which are used in different combinations (1 to 4 times or more daily) [3]. Table 1 lists the different insulin preparations together with their durations of action.
Table 1: Durations of action of different insulin preparations.
View Table 1
Disadvantages of current approved insulin regimens relate to the injectable mode of its administration. Daily injections have led to non-compliance, which has obvious effects on patient outcomes. In addition, parenteral administration bypasses the liver, unlike physiologically-secreted insulin. Hepatic first-pass metabolism, which decreases the amount of circulating insulin by approximately 50% in normal individuals, prevents peripheral hyperinsulinaemia and its associated complications [5-11].
Advances in Insulin Therapy
The recent advances in novel insulin therapy for diabetes include newer injectable formulations, artificial pancreas systems, oral,transmucosal, and transdermal dosage forms. These are covered in more detailunder the following subheadings and aresummarizedin Table 2.
Table 2: Newer insulin formulations and details of their respective experimental results.
View Table 2
Injectable insulin: Newly discovered parenteral insulin formulations include a different insulin glargine concentration of 300 U/ml, an ultra-long acting insulin degludec, as well as ultra-short acting insulin Linjeta™. Both formulations show favourable characteristics that may replace or supplement currently available insulin injections.
Insulin glargine 300 U/ml (IGlar-300): 2 randomized controlled trials compared the efficacy of 2 different doses of insulin glargine, 300 U/ml (IGlar-300) and 100 U/ml (IGlar-100), on patients with T2DM. One study involved T2DM patients using basal and mealtime insulin (EDITION 1 study) [12], whereas another studied the outcomes on T2DM patients using basal insulin and oral antihyperglycaemic drugs (EDITION 2 study) [13]. Both studies showed that IGlar-300 is as effective as IGlar-100 in terms of HbA1C reduction, but the former is associated with a lower risk of nocturnal hypoglycaemia. The EDITION 1 study found a 10% absolute and 21% relative risk reduction of nocturnal hypoglycaemia, with no increase in daytime hypoglycaemia [12]. Similarly, the EDITION 2 study demonstrated a 23% risk reduction in nocturnal hypoglycaemia (P=0.038), in addition to decreased risk of hypoglycaemia at any time (24 hours) [13].
Insulin degludec (IDeg): A new formulation of modified human insulin with ultra-long action, with a half-life exceeding 24 hours [15,21,29]. This novel preparation has been compared with the current basal insulin regimen, insulin glargine (IGlar). Studies show that IDeg, when compared to IGlar, resulted in similar, or even better glycaemic control, with less hypoglycaemic episodes and lower risk of nocturnal hypoglycaemia [5,14-20]. Phase 3 clinical trials on T1DM and T2DM patients indicated that IDeg was similar in efficacy to IGlar in reaching a target HbA1C of 7%, whilst reducing the risk of nocturnal hypoglycaemia [30]. In addition, IDeg resulted in a greater improvement in overall health status over 2 years [22], with similar HbA1C control [17], and required lower doses for glycaemic control [19], when compared to IGlar. Although IDeg may require less frequent injections (three times a week) compared to IGlar (once daily) in order to maintain effective basal insulin levels [5,23], more recent studies in T2DM patients do not recommend this thrice weekly IDeg regimen, as it may result in higher risk of hypoglycaemia [24]. However, the time taken to recover from hypoglycaemic episodes back to normal glucose levels was similar in both IDeg and IGlar [25]. Compared to insulin detemir (IDet), IDeg also fared better in terms of glycaemic control, less nocturnal hypoglycaemia and less frequent injections, when used in a basal bolus regimen with insulin as part [5,26,31]. Due to the slow release and achieving a steady-state profile in once daily dosing, IDeg allows for flexibility in timing of doses, without affecting efficacy and safety. This is particularly important to patients who are elderly, with learning difficulties, or those depending on healthcare professionals for insulin injections [29,30]. However, IDeg has not been approved by the Food and Drug Association (FDA) due to facts indicating an increase in the risk for major adverse cardiovascular events (MACE) associated with IDeg [5,27,28].
Linjeta™ (previously VIAject™): This form of recombinant human insulin has an ultra-short action, with faster onset compared to human soluble insulin and insulin lispro (LIS) [5,32,33], and showed less within-subject variability compared to regular human insulin (RHI) [5,34]. When compared to RHI and LIS, Linjeta™ exhibited less postprandial glycaemic excursions and oxidative stress, as well as improved endothelial function [35,36].
Artificial pancreas
Also known as an integrated closed-loop control (CLC), this system utilises real-time feedback from continuous glucose monitoring (CGM), to continuously adjust insulin administration via an insulin pump (continuous subcutaneous insulin infusion, CSII) depending on blood glucose levels [102]. The system consists of a continuous glucose monitor, linked wirelessly to an insulin infusion pump, which delivers insulin in accordance to an algorithm, automatically. This method mimics physiological pancreatic β-cell function to a certain extent, and reduces the risk of hypoglycaemia [5]. It is minimally invasive as the electrode is implanted in the subcutaneous tissue and measures glucose electrochemically [103].
However, this system has limitations due to delays in both insulin action and blood glucose sensing. First, the sensors measure the glucose in the interstitial fluid rather than blood (a delay of 5-15 minutes), which can lead to errors in accuracy? Second, insulin analogues available today have a lag period in their absorption into the bloodstream and, thus, in their onset of action. These may result in insulin action to be delayed by approximately 20 and 100 minutes in peripheral tissues and the liver, respectively. The algorithm used by the system can help circumvent this delay to some extent. Previous proportional-integral-derivative (PID) control algorithms merely respond to glucose levels, and faced the previously mentioned lag periods. The newer model-predictive control (MPC) algorithm models the patient's metabolic system and reduces this problem by planning the insulin delivery several moves ahead. This 'smart' system suffers less lag periods and extreme blood glucose fluctuations [5,37,38].
There are numerous advancements in this artificial pancreas system to date. The development of rapid-acting insulins such as Linjeta™, and absorption enhancers may help in improving the response time of CLC systems [5]. One such enhancer is a recombinant insulin hyaluronidase (rHuPH20), which improved glucose control after meals and reduced hypoglycaemia in patients with T1DM and T2DM [5,104,105]. Additionally, it has been shown that the control system for insulin delivery can be programmed into a regular smart phone [106,107]. This may make this system less costly, more accessible and more easily upgradable. Studies in outpatient settings with artificial pancreas systems found that the current settings are safe and efficient for day-to-day use. With advancements in technology, even better outcomes can be expected in the future [39].
Oral insulin
The ultimate goal of exogenous insulin therapy is via oral administration, due to its numerous advantages. Firstly, oral delivery of insulin enters the portal circulation and reaches the liver before entering the systemic circulation. This resembles the pathway of physiologically secreted insulin, resulting in inhibition of glucose production by the liver and avoidance of peripheral hyperinsulinaemic effects [5,6,8-11]. Secondly, the inconvenience of invasive (injectable) forms of insulin has led to problems in patient compliance, ultimately affecting patient outcomes [5,7,10,108].
Nevertheless, this ideal insulin formulation has so far been elusive due to the difficulties faced in designing such a delivery system. Orally administered drugs need to survive harsh gastric conditions in which they are exposed to acidic and enzymatic degradation. Subsequently, intestinal conditions further expose the drugs to a myriad of digestive enzymes [5,6,10,109-112]. The drugs that survive these conditions then need to penetrate the mucus layer overlying the absorptive surfaces of the gastrointestinal tract (GIT) and pass through the epithelial cells to enter the blood stream. Insulin, being a peptide molecule, is easily degraded by the conditions in the GIT, which significantly lowers the bioavailability of orally administered insulin [5,6,10].
One method of circumventing this difficulty would be to design an insulin delivery system that would protect it from damage throughout its GIT transit and release the insulin at the absorption sites. Nanotechnology has shown promising results in the design of potential oral insulin nanocomposites. These delivery systems can be designed to protect the insulin molecules from degradation, modify the release profile of insulin from the nanoparticles (NP), and/or target specific absorption sites, ultimately increasing the bioavailability of orally delivered insulin [10].
We have published a review article on current nanodevices for targeted oral insulin therapy in the Journal of Nanomedicine and Nanotechnology [10]. These nanoformulations include those based on chitosan (CS) and CS-derivatives, poly(lactide-co-glycolide) (PLGA), acrylic-based NP, poly(lactide) (PLA), poly(e-caprolactone) (PCL), poly(allylamine), dextran, lipid-based formulations such as liposomes and solid-lipid nanoparticles (SLN), as well as multi-layered NP. In vitro and in vivo studies on normal and diabetic mice/rats found evidence to indicate that these NP formulations improved insulin absorption and bioavailability via the oral route, resulting in a greater hypoglycaemic effect. Some NP preparations caused a prolonged hypoglycaemic effect as well [10]. We have also studied another inorganic NP known as carbonate-apatite (CO3Ap), which has the potential to be used as a delivery device to deliver insulin via the oral route. Preliminary data suggests that the insulin-CO3Ap composites can be made more stable by incorporation of strontium ions [113].
More complicated nanoformulations have also been experimented upon. For example, nanolayer encapsulation of insulin-CS complexes has been studied for the sustained release of insulin after oral administration. The insulin-CS microparticulate cores were coated with CS/heparin multilayer coatings, with poly(ethylene) glycol (PEG) present in the precipitating and coating solutions. This preparation improved insulin loading capacity (>90%), and increased insulin stability (74% decrease in solubility at acidic pH in vitro), relative to non-encapsulated insulin. In vivo studies on streptozotocin (STZ)-induced diabetic mice found that this delivery system caused a drop in fasting glucose levels by up to 50%, with a sustained and dose-dependent behaviour. The formulation also decreased the surge in blood glucose levels post-meals, without risk of hypoglycaemia [40]. A separate nanoparticulated insulin in multiple emulsion form also showed promise for oral delivery. Insulin-polymeric NP made using layer-by-layer adsorption method, which is then incorporated into an emulsion, forming a nanoparticulated multiple emulsion, consisting of insulin nanoaggregates between 300 to 400nm in size. These nano-insulin preparation exhibited good release characteristics and offered protection to insulin at different pH levels [41]. Insulin-loaded alginate/CS blend gel beads cross-linked by glutaraldehyde showed favourable characteristics in simulated gastric fluid (SGF) and simulated intestinal fluid (SIF). These beads were prepared by dripping a homogeneous solution of alginate and CS into 2% calcium chloride solution, forming calcium cross-linked beads, which in turn, were immersed in 2% glutaraldehyde solution. These dual cross-linked alginate/CS beads exhibited greater stability in SGF and SIF. Due to the cross-linking, the presence of CS was found to reduce the cumulative insulin release in SGF and improved the performance of the alginate/CSbeads SIF [42].
These previously-mentioned studies were limited to in vitro and in vivo experiments. However, a new insulin analogue known as IN-105 is currently being studied in phase 2 trials, by the pharmaceutical company Biocon, in association with Bristol-Myers Squibb [5,114]. IN-105, a conjugated recombinant insulin [115], lasted longer in the GIT, had better absorption, was less immunogenic and less mitogenic compared to insulin, but exhibited similar pharmacological activity. IN-105 also did not show significant genotoxic, mutagenic or teratogenic potential [5], and may have a wide therapeutic window as study subjects showed no clinical hypoglycaemic effects [43]. It also showed a dose-dependent effect on 2-hour postprandial glucose levels [5,43,44].
Transmucosal insulin
Transmucosa insulin delivery include buccal, pulmonary (inhaled), nasal, ocular, as well as rectal routes. These routes are common in that they involve insulin absorption/transport across mucosal surfaces into the bloodstream.
Buccal insulin: Buccal delivery of insulin involves aerosol delivery of the drug into the oral cavity, after which absorption occurs through the inner surfaces of the cheeks and the back of the mouth. This distinguishes it from oral delivery, which is absorbed further along the GIT, as well as pulmonary delivery, which is absorbed in the lungs [5]. The buccal mucosa is relatively immobile, highly vascularised, and recovers quickly after exposure to stress. These characteristics allow administration of drugs in retentive dosage forms. Additionally, the molecules absorbed via the buccal route enter the internal jugular vein, and thence into the systemic circulation, bypassing hepatic first-pass metabolism, leading to improved bioavailability. Furthermore, buccal administration is convenient, painless, and easily accessible [48,116,117].
Problems faced with this route of administration include saliva flow as well as the multi-layered epithelial surface of the oral cavity [5,48]. As with oral insulin, nanoparticles (NP) have been shown to facilitate buccal administration [5]. Absorption enhancers (e.g. bile acid salts, sodium lauryl sulphate, etc.) can aid buccal delivery by increasing permeability of the buccal epithelium, or by inhibiting enzymatic action to improve stability of drug molecules. However, these enhancers present additional side effects, such as mucosal irritation as well as having an offensive taste. In particular, bile acid salts, one of the most effective enhancers, have a bitter taste [48].
Insulin-coated NP embedded in polymeric films showed promising results in enhancing insulin transport though a cultured tri-dimensional model of human buccal mucosa (EpiOral). The cationic polymer used is a polymethacrylate derivative, known as trimethylammonioethyl methacrylate chloride, Eudragit® RLPO (ERL) [46]. Another formulation recently studied involved insulin-loaded NP embedded into chitosan films. This preparation improved insulin absorption through the same EpiOralbuccal mucosa model by 1.8 times, compared to pure insulin [47].
Vesicles can be utilised to improve insulin delivery through the buccal route. A new form of deformable lipid vesicle known as a transferosome was found to improve buccal absorption of insulin in rabbits. These transferosomes consist of soybean phosphotidylcholine, cholesterol, and sodium deoxycholate (NaDC). Experimental results indicate relative pharmacological activity and relative bioavailability of 15.59% and 19.78%, respectively, compared to SC administration of insulin solution. Transferosome delivery of insulin was also found to be better than delivery by conventional insulin vesicles [48,50].
In addition, many studies have tested the effect of absorption enhancers to improve buccal insulin delivery. One such formulation is a combination of soybean lecithin and propanediol, which was shown to enhance buccal insulin delivery in vivo, with significant hypoglycaemia lasting 4-5 hours [5,48,51,118]. Another experiment studied a Pluronic F-127 (PF-127) gel containing unsaturated fatty acids. Three types of fatty acids were tested, namely oleic, eicosapentaenoic and docosahexaenoic acids, all of which improved insulin release, caused continuous hypoglycaemic effects in normal rats, with oleic acid giving the highest pharmacological availability of 15.9 ±7.9% [48,52]. Another compound, lysalbinic acid is also known to increase the permeability of buccal mucosa to certain peptides including insulin, and studies on hamster cheek pouch models have proven this [48,53,54].
Mucoadhesive delivery systems allow improved buccal absorption without the side effects associated with absorption enhancers. These formulations result in interactions between the delivery system and the mucosa, increasing the residence time of drug molecules at the absorption sites [48,119]. NP with mucoadhesive properties were also studied for buccal insulin delivery. These pelleted bioadhesive polymeric NP containing insulin resulted in significant hypoglycaemia in diabetic rats after 7 hours, with no risk of hypoglycaemia [48,49].
Oral-lyn™ is a market available buccal insulin preparation developed by Generex Biotechnology Corp. for patients with T1DM and T2DM. It is delivered via the company's RapidMist™ hand-held device, which allows buccal absorption and prevention of deposition in the lungs. Oral-lyn™ consists of a mixed micellar solution containing insulin and absorption enhancers. Each puff delivers 10U insulin, and with an absorption rate of 10%, results in 1 U of insulin absorbed [5,44,45,48]. Clinical trials involving individuals with T1DM, T2DM, as well as healthy subjects found that Oral-lyn™ showed a dose-dependent absorption profile, with faster onset and shorter duration relative to SC regular insulin [5,45].
Pulmonary (inhaled) insulin: Inhaled drugs are absorbed into the alveolar-capillary network, which has the advantage of having a large surface area (~140m2), thin diffusion barrier (~0.1-0.5mm), as well as being non-invasive. Drugs can be aerosolised and delivered with a median aerodynamic diameter smaller than 5µm, effectively targeting the alveoli as sites for absorption. Pulmonary delivery bypasses digestive enzymes and first-pass metabolism associated with oral delivery. Although enzymes are present in the lungs, they are involved in different metabolic pathways than those in the GIT [48,63,120].
Insulin microcrystals with a mean diameter of 3μm were prepared and administered to STZ-induced diabetic rats by using a sieve-type ultrasonic nebuliser. Prolonged hypoglycaemic effects were observed over 7 hours. This may be explained by a sustained-release profile of insulin from the microcrystals that were deposited throughout the lungs [48,57]. Further studies on insulin microcrystals found that the addition of zinc caused an enhancement of the hypoglycaemic effect in rats (17% minimum reductions in blood glucose) [48,56].
Hyaluronic acid (HA) exhibits valuable properties to aid in pulmonary insulin delivery, including its mucoadhesivity, which prolongs contact of the drug delivery system with absorption sites in the lungs, improving insulin absorption. In vivo studies on beagle dogs suggested that the addition of zinc ions (Zn2+) or hydroxypropyl cellulose (HPC) to insulin/HA dry powder system improved the mean residence time of the preparation by >9 times and >7 times, respectively, as compared to spray dried pure insulin [48,58].
A number of absorption enhancers have been experimented upon, to improve insulin delivery via the pulmonary route. In vitro and in vivo studies suggested that phospholipids enhance pulmonary absorption of insulin. When 1,2-dipalmitoyl phosphatidylcholine (DPPC) was physically mixed with insulin and administered to anaesthetised rats, greater hypoglycaemic effects were seen compared to insulin and liposome mixture [48,59]. Another study investigated the effect of additives to insulin absorption. A dry powder form of insulin was found to have a higher bioavailability than a pH 7.4 insulin solution. Bacitracin (1.4mg/dose) and Span 85 (1mg/dose) improved insulin (solution form) bioavailability to almost 100%, but are not effective in dry powder forms. However, citric acid added to insulin dry powder increased the hypoglycaemic effect, with bioavailabilities of 42% and 53% for dry powders containing 0.025 and 0.036mg/dose citric acid, respectively. Moreover, citric acid was not found to cause acute toxicity to lung cells [48,60]. Another group of absorption enhancers belong to the family of cyclodextrin (CD) derivatives, namely tetradecyl-β-maltoside (TDM) and dimethyl-β-cyclodextrin (DMβCD), which have been tested on anaesthetised rats. The relative bioavailability of insulin preparations with TDM was higher (0.34-0.84%) than those containing DMβCD (0.19-0.48%), indicating that TDM is a more effective absorption enhancer. It was also found that both agents have reversible effects on the respiratory epithelium, which was restored 120 minutes post-exposure [48,61]. Hydroxy-methyl-amino-propionic acid (H-MAP) was investigated as a potential pulmonary absorption enhancer in fasted anaesthetised rats. It was discovered that co-administration of H-MAP with insulin affects pharmacokinetics and pharmacodynamics in a dose-related manner, with maximum effects at 16mg/kg
H-MAP and 1.3U/kg insulin. At these doses, there was a >2.5-fold increase in relative insulin bioavailability, achieving twice the maximum insulin concentration, with twice the reduction of blood glucose, compared to the same dose of insulin alone [62]. Liposomes are another method to improve pulmonary transport of insulin. Studies have found that liposomes can be made and insulin encapsulated (and remained stable) within them. In vivo studies in alloxan-induced diabetic mice indicated that aerosolised insulin-liposomes were homogeneously distributed throughout the alveoli and drug retention times were increased, causing a measurable hypoglycaemic effect [48,63]. Another particulate formulation combines calcium phosphate (CAP) and polyethylene glycol (PEG) to make suspensions of insulin-CAP-PEG particles. Pharmacokinetic analyses indicated that insulin had a longer half-life and residence time when given to fasted rats in CAP-PEG particles compared to in solution. Spray instillation was also found to be more efficient than intratracheal instillation to deliver insulin method via the pulmonary route [48,64].
Nanoparticulate delivery systems have helped improve pulmonary insulin delivery as well. One such formulation involves insulin-loaded poly(lactide-co-glycolide) (PLGA) nanospheres with a mean diameter of 400nm, prepared by modified emulsion solvent diffusion method in water. These NP were prepared as aqueous dispersions (6mg/ml), nebulised by a sieve type ultrasonic nebuliser, and administered to fasted guinea pigs through a spacer by using a constant volume respirator for 20 minutes. Administration of 3.9IU/kg insulin caused substantial prolonged hypoglycaemic responses over 48 hours, in contrast to 6 hours by nebulised aqueous insulin solution. This suggested that insulin was released in a sustained manner from PLGA nanospheres that were distributed throughout the lung [48,65]. Insulin loaded in polybutylcyanoacrylate NP prepared by emulsion polymerisation also improved bioavailability in studies on normal rats. Intratracheal administration caused significant hypoglycaemic responses, with minimum blood glucose concentrationsof46.9%, 30.4% and 13.6% of initial levels after administration of 5, 10 and 20 IU/kg insulin (in polybutylcyanoacrylate NP), respectively [48,66].
A recently FDA-approved product called Afrezza® has been developed by MannKind Corp. This rapid-acting insulin is delivered as an inhalational powder before meals. Studies to assess its safety and effectiveness were carried out in 3017 participants (1026 with T1DM, 1991 with T2DM). When Afrezza® was used as part of the basal-bolus regimen with basal insulin in T1DM patients, HbA1C reduction in 24 weeks was acceptable but not as much as that achieved by insulin as part. When used in combination with oral hypoglycaemic drugs in T2DM patients, Afrezza® achieved a greater reduction in HbA1C after 24 weeks (compared to placebo) [55].
The ideal pulmonary delivery system would have good insulin-loading efficiency, avoidance of insulin break down, predictable and reproducible release profile, as well as minimal side effects. These are the challenges faced by researchers searching for an inhaled form of insulin therapy. It is important to consider the adverse effects of inhaled insulin including the greater risk of hypoglycaemia, greater weight gain, and mild to moderate cough in 25% of patients [48,121-123]. Insulin doses are also dependent on patient co-morbidities, as smokers and asthmatics require lower and higher doses, respectively [48,124,125]. Furthermore, to achieve a comparable glycaemic response, inhaled insulin needs to be given at much higher doses compared to SC administration [48,126,127]. There is also the issue of insulin-directed antibodies [48,128]. Nevertheless, inhaled insulin appears to be a reasonable alternative to short-acting insulin injections in diabetic patients [48,126,129].
Nasal insulin: The nasal cavity is lined by an epithelial layer that has a large surface area due to the existence of microvilli on the epithelial cells. Together with the high total blood flow and porous endothelial membrane, this facilitates absorption of molecules into the subepithelial capillary beds, directly into the general circulation. This route also bypasses the liver and avoids first-pass metabolism. All together, these can allow fast absorption (comparable to intramuscular or even IV injections) and onset at lower doses, and fewer side effects. Nasal administration is also convenient and less invasive, which can lead to better patient compliance [48,76,130,131]. Administering intranasal insulin post-meals result in pulsatile increases in blood insulin levels, mimicking normal physiological secretion. Considering all these advantages, intranasal insulin has great potential to be used in the treatment of patients with T1DM and T2DM [48,130].
Difficulties faced by nasal drug delivery include mucociliary clearance, enzymatic activity, as well as the epithelial lining itself that prevents passage of peptide molecules with high molecular weights and hydrophilic natures. Nasal bioavailability can be increased by use of absorption enhancers, proteolytic enzyme inhibitors, mucoadhesive formulations, as well as dry powder systems to deliver insulin via the nasal route [48,132].
One study investigated the potential use of alkylglycosides as absorption enhancers for the nasal absorption of insulin, as in vivo studies in rats made hyperglycemic by xylazine/ketamine anesthesia. When drops containing 2U insulin and 0.03-0.5% alkylglycoside were instilled, rapid hypoglycaemic effects were observed, together with increased levels of immune reactive insulin in the blood, with maximal hypoglycaemia between 60-120min post-administration. Dodecylmaltoside, tridecylmaltoside, tetradecylmaltoside and dodecylsucrose (a compound that differs from dodecylmaltoside by only one carbohydrate residue) effectively enhanced insulin absorption. However, nonylglucoside needed higher concentrations of 0.25-0.5% to behave as an effective absorption enhancer [68]. Further experiments on glycosides, made up to maltose or sucrose linked to longer alkyl side-chains (C13-16), were tested on anaesthesised rats, and their efficacy as absorption enhancers were compared with previously tested alkylglycosides with shorter alkyl side-chains (C8-12). Results indicate that for alkylmaltoside derivatives, tetradecylmaltoside (C14) caused maximal enhancement in insulin absorption. Longer-chained pentadecylmaltoside (C15) and hexadecylmaltoside (C16) were less potent and required higher concentrations for maximal effect. On the other hand, for alkanoylsucrose derivatives, tridecanoylsucrose (C13) and tetradecanoyl sucrose (C14) were most potent. In addition, tetradecyl maltoside increased insulin absorption even when administered 15 minutes before insulin. It was determined that the potency of the enhancers depended more on the alkyl side-chain length rather than the glycoside moiety [48,67].
Emollients such as sucrose cocoate (SL-40), which is a mixture of sucrose esters of coconut fatty acids in aqueous ethanol solution, has also been tested as a absorption enhancer for nasal insulin administration in vivo on anaesthetised Sprague-Dawley rats. A 0.5% sucrose cocoate formulation increased nasal insulin absorption, with resulting hypoglycaemic effects. Mass spectrometry identified sucrose monododecanoate as the most common ester in the sucrose cocoate mixture [69].
Mucoadhesive delivery systems can aid in improving bioavailability by countering the nasal mucociliary clearance mechanism. These systems prolong drug residence times at absorption sites and protect the drug molecules from enzymatic degradation [48,133,134]. These include gels, liposomes and microspheres, which absorb water from the mucus layer lining the nasal cavity and forming a gel-like layer over the nasal mucosa. In addition, the absorption of water causes the nasal epithelial cells to be dehydrated, resulting in the separation of tight junctions between cells, increasing their permeability to drugs via paracellular absorption [48,73,135]. An example of a gel preparation is a carbopol-based nasal gel spray, containing insulin, which caused a significant drop in blood glucose levels in rabbits. The bioavailability of insulin administered in the nasal gel preparation was 20.6% compared to IV insulin [48,70]. Another insulin gel formulation uses bioadhesive chitosan (CS) gel containing 4000 IU/dl insulin, administered to diabetic rabbits. Results show that gels made up of 2% medium molecular weight CS with ethylenediaminetetraacetic acid (EDTA) enhanced insulin absorption and caused a drop in glucose level by up to 46% of that caused by IV insulin [48,71].
Microsphere preparations for nasal insulin include insulin/CS microspheres made by emulsification-cross linking process. In vivo studies on diabetic rats found that microspheres with 400mg CS and 70mg ascorbylpalmitate (used as a cross-linker) resulted in an absolute bioavaliability of 44%, with a corresponding 67% decrease in blood glucose compared to IV insulin [48,72]. A separate study tested aminatedgelatin microspheres (AGMS) to deliver intranasal insulin to rats. Fluorescein isothiocyanate (FITC)-labelled insulin was used in this study, which was released slower from AGMS (cumulative release of 18.4% within 30min, 56.9% within 8h), compared to native gelatin microspheres (GMS) (cumulative release of 32.4% within 30min, 75.1% within 8h). Additionally, AGMS increased nasal insulin absorption significantly when given in dry powder form. It was suggested that AGMS works by absorbing water from the nasal mucosa, temporarily dehydrating the epithelium, hence, opening tight junctions. The cationic nature and mucoadhesivity of AGMS also contributes to its absorption enhancing effects [48,73]. A separate microparticle-based delivery system using a thiolated chitosan conjugate, known as chitosan-4-thiobutylamidine (CS-TBA) was tested to deliver insulin nasally in rats. The nasal preparation consisted of FITC-labelled insulin, CS-TBA and reduced glutathione (as a permeation mediator). The insulin showed a controlled-release profile over 6 hours, with an absolute bioavailability of 7.24%, which is higher compared to using unmodified CS [48,74,75].
Nanotechnology has opened up new pathways in researching novel nasal insulin delivery systems. One formulation tested insulin/CS NP prepared by ionotropic gelation of CS glutamate and tripolyphosphatepentasodium, and by simple complexation of insulin and CS, but found that the enhancing effect was not as great as CS in powder form [48,136]. Another formulation involves a CS derivative: PEGylated trimethyl CS (TMC) nanocomplexes (NC). These insulin/PEGylated TMC NC managed to reduce blood glucose levels by 34-47% in anaesthetised rats, were found to have less toxic effects on nasal epithelium compared to the non-PEGylated counterparts, suggesting its suitability to be used as a nasal insulin delivery system [76]. Another set of NC tested for this same purpose were made from amine-modified poly(vinyl alcohol)-graft-poly(L-lactide), and prepared by spontaneous self-assembly of insulin and the water-soluble amphiphilic polymer. In vivo studies on fasted healthy rats, found that the NC decreased blood glucose levels by 50% (from basal levels), 50 to 80 minutes post-administration, and studies on STZ-induced diabetic rats observed a 30% decrease in blood glucose within 75-95 minutes. There was no evidence of nasal mucosal damage 4 hours post-administration, as confirmed by histological examination [77]. An amphiphilicglycopolymerpoly(2-lactobionamidoethyl methacrylate-random-3-acrylamidophenylboronic acid) (p(LAMA-r-AAPBA)) was used in the manufacture of NP to deliver insulin intranasally. It was demonstrated that modification of the glycopolymers affects insulin release in vitro. Further tests show good cytocompatibility, with NP internalisation into Calu-3 cells via clathrin-mediated and lipid raft/caveolae-mediated endocytosis. In vivo studies on diabetic rats show significant hypoglycaemic effect after nasal administration [78].
Nasally delivered insulin seems to be a possible non-invasive method of administering insulin. However, there are certain problems that need to be addressed. There are large amounts of intra- and inter-individual variability in bioavailability, as well as limitations to the amount of insulin that can be administered for each nasal instillation. More importantly, the preparation must be safe to be used in the long run, as insulin is used in long-term diabetic management. Nasal irritation and possible damage to the nasal mucosa and ciliary function are concerning drawbacks [48]. Absorption enhancers are useful to increase nasal insulin bioavailability, but are also associated with adverse effects, especially at effective concentrations and/or prolonged use. Surfactants and bile salts, popular absorption enhancers, can cause irreversible nasal mucosa damage [48,137-139]. Another potent enhancer is dimethyl-β-cyclodextrin (DMβCD), a cyclodextrin derivative, which was shown to increase insulin absorption via the nasal route in rats at a concentration of 5% (w/v), with a bioavailability of almost 100%. However, in vitro studies show that DMβCD affects ciliary movement [139]. Hence, further studies are needed before nasal insulin delivery systems are ready for clinical use.
Ocular insulin: Insulin can also be made into eye drops to allow non-invasive administration. This route is also relatively convenient and allows rapid systemic absorption, bypassing the GIT and liver. Moreover, tissues in the eye are less likely to develop immunological reactions, compared to other tissues. This is supported by a 3-month study on subjects that was administered daily insulin eye drops, and found no ocular side effects or tolerance [48,140]. Problems with this route of delivery are local irritation, as well as loss of drug molecules via blinking, tearing and drainage [48]. Insulin delivered as eye drops in a randomised, placebo-controlled trial was shown to not cause any clinically detectable toxic effects to the anterior eye structures in healthy human volunteers (in single doses up to 100 U/mL). However, results of this study also indicated that there was no significant systemic absorption of insulin without any effect to blood glucose levels [48,141]. A separate study on rats also showed insignificant insulin absorption when applied as eye drops as a solution in normal saline. When emulsants were added (e.g. saponin, Brij-78, BL-9, and several alkylglycosides), insulin absorption improved and hypoglycaemia was observed [142].
Due to the limited bioavailability of insulin delivered through the ocular route, numerous absorption enhancers have been experimented upon. Fusidic acid and glycocholate were proven to increase ocular insulin absorption in rabbits, especially when administered at higher pH levels (pH 8 as opposed to pH 3.5) [81]. In a study on albino rabbits, 1% concentrations of polyoxyethylene-9-lauryl ether (POELE or BL-9), sodiumglycocholate (NaGC), sodiumtaurocholate (NaTC), and sodiumdeoxycholate (NaDC) were each tested as absorption enhancers, and it was found that hypoglycaemia was possible with ocular insulin preparations. The bioavailabilities ranged from 3.6-12.6% with absorption enhancers, as compared to 0.7-1.3% without enhancers. However, systemic absorption of insulin was contributed by the nasal mucosa more than by the conjunctival mucosa by about 4 times [48,79].
Alkylglycosides have also been shown to enhance systemic absorption of insulin delivered via ocular delivery. Studies on rats show that tetradecyl-, tridecyl- and dodecylmaltoside as well as dodecylsucrose showed effective improvements in insulin absorption, at concentrations as low as 0.125%, as compared to NaGC (a bile salt), which is only effective at concentrations over 0.5%. The best absorption enhancers were the alkylglycosides with the greatest hydrophobic properties [48,80]. Studies on healthy dogs and cats have also been carried out to investigate a variety of absorption/permeation enhancers, namely saponin, POELE (BL-9), polyoxyethylene-20-stearyl ether (Brij-78), fusidic acid, dodecylmaltoside, and tetradecylmaltoside [48,82,83]. In dogs, 0.5% saponin, 0.5% and 1% POELE (BL-9), 0.5% and 1% dodecylmaltoside, as well as 0.5% and 1% tetradecylmaltoside significantly enhanced insulin absorption with corresponding glycaemic effects [48,83]. In vivo studies in cats showed that substantial increase in insulin absorption and hypoglycaemic effects were noted when insulin was administered with 0.5% saponin, 0.5% Brij-78, as well as 1% and 2%POELE (BL-9) [82]. In contrast, ocular delivery of native insulin had nosignificant effects in both dogs and cats, insulin delivered with 0.5% Brij-78 and 0.5% fusidic acid had no significant effects in dogs, and insulin delivered with Brij-99 had no significant effects on cats [82,83].
In a separate study, insulin-loaded cationic liposomes were prepared to deliver and prolong insulin retention time in the precorneal area of the eye, which produced hypoglycaemic effects in rabbits [48,84,143]. Liposomes made up of egg phosphatidylcholine with cholesterol and stearylamine (10:2:1 in weight ratio), loaded with insulin, resulted in significant hypoglycaemic effects in normal rabbits 90-120 minutes post-administration [84].
Absorption enhancers may cause adverse effects; hence, these compounds were also tested for possible unwanted reactions in test subjects. Saponin (0.5% conc.) caused temporary irritation to the eyes, whereas Brij-78 and POELE (BL-9) were well tolerated in studies on dogs and cats [82,83]. Fusidic acid, dodecylmaltoside, and tetradecylmaltoside showed no side effects in dogs [83]. In vivo studies on POELE (BL-9) and Brij-78 on rabbits as permeation enhancers for ocular insulin administration, showed no local side effects or allergic response after 3 months of twice daily ocular instillations. Uptake and efficacy of insulin also remained constant throughout the study period [48,144].
Gelfoam®, a commercially available absorbable gelatin sponge, has been used in studies to fabricate an ocular device for the delivery of insulin. In vivo studies achieved prolonged insulin delivery, especially with the addition of Brij-78 as an absorption enhancer. There were no risk of hypoglycaemia, and near normal glucose levels were achieved within 60 minutes after the removal of the insert [48,85,86]. Due to the potential side effects of absorption enhancers, the role of acid in insulin delivery via Gelfoam® devices, without absorption enhancers was investigated. Results suggest treatment of Gelfoam® devices with either 5% acetic acid or 1% hydrochloric acid (HCl) was sufficient to improve systemic absorption of insulin [48,87-89]. In vivo studies on rabbits show that 0.2mg of insulin in a single Gelfoam® device managed to lower blood glucose levels to 60% of initial levels over 8 hours, and no eye irritation was caused by Gelfoam® devices treated with acetic acid up to 30% concentration [89].
Insulin delivered via the ocular route seems possible with no significant toxicity, as suggested by preliminary studies. However, it is important to note that intraocular devices presents with a variety of complications, partly due to the fact that it needs to remain in place for a few hours. This makes local irritation effects more problematic [48]. It has also been suggested that insulin might have metabolic and/or mitogenic effects on the ocular surface in humans [145]. Comfort is another issue, as well as ensuring the device does not get dislodged during daily activities as well as during sleep. Finally, it is important that the release of insulin from ocular devices is constant and reproducible. As of yet, further studies need to be carried out before ensuring that ocular insulin can be used clinically in diabetic patients [48].
Rectal insulin: This route involves absorption in the GIT, but differs from the oral route as it bypasses the more proximal areas that are actively involved in digestion, as well as being relatively unaffected by diet and gastric/intestinal motility. Hepatic first-pass metabolism may also be avoided to a certain extent as there are porto-systemic anastomoses in rectal vessels. These vessels connect the portal system to the systemic system, hence allowing absorbed drugs to directly enter the systemic circulation [48].
In animal studies, suppositories were formulated with 50 U insulin and either 50mg deoxycholic acid (DCA), sodium taurocholate (NaTC), or both, and tested on alloxan-induced hyperglycemic rabbits. These resulted in relative hypoglycaemic effects of 38.0%, 34.9%, and 44.4%, respectively, compared to SC insulin (40 U). When 50mg polycarbophil was added to the preparation containing insulin, DCA and NaTC, the relative hypoglycaemia increased to 56%. Therefore, approximately 50% of the efficacy of SC insulin can be achieved with rectal insulin formulations [48,90]. Studies on diabetic beagle dogs found that 50mg sodium salicylate or 1% POELE (BL-9) enhanced insulin absorption from suppositories (containing 5 U /kg insulin and Witepsol W35 as a base), producing a relative hypoglycaemic effect of approximately 49-55% (compared to SC insulin 4 U/kg) [48,91]. Similar hypoglycaemic effects (approximately 50% relative to SC insulin) were achieved with insulin-loaded Witepsol W35-based suppositories containing either sodiumdeoxycholate (NaDC) plus sodium cholate (NaC) (100mg/50mg, respectively), sodium taurodeoxycholate (NaTDC) (100mg), or sodium taurocholate (NaTC) (100 mg) as rectal absorption enhancers. Adjusting the insulin dose in the rectal formulation in accordance to the degree of hyperglycaemia allows a desirable hypoglycaemic effect [48,92]. Gel formulations have also been tested for transrectal insulin delivery. A study on rabbits utilised CS gel as a carrier, with dimethyl-β-cyclodextrin (DMβCD) as absorption enhancers. Prolonged insulin release was achieved by the CS gel, and maximum hypoglycaemic effects were seen in a formulation containing insulin-CS gel with 5% DMβCD. This study found that such a delivery system is able to improve insulin delivery transvaginally as well [93].
Suppositories containing 100U insulin and 200mg sodium salicylate as an absorption enhancer were tested in human studies (4 healthy, 15 T1DM individuals). Hypoglycaemic effects were achieved by 15 minutes, and lasted up to 90 minutes post-administration [94]. Another human study experimented on insulin suppositories containing 200U insulin with 100mg sodium cholate (NaC), which produced a maximum of 47.7% reduction in blood glucose levels (90min post-administration) compared to 50.6% with SC insulin (20U). This preparation managed to avoid significant postprandial rise in blood glucose in 7 T1DM patients, and were found to be safe and well-tolerated. However, when tested on healthy control subjects, it caused severe hypoglycaemia 1 hour post-administration [95].
An additional technique to improve rectal absorption of insulin is by creating an adhesive interaction between the delivery system and the rectal mucosa, increasing drug residence time at the absorption sites. This mucoadhesive concept has been tested with a thermo-reversible insulin liquid suppository, which at body temperature, undergoes a phase transition to form bioadhesive gels. Insulin bioavailability was improved with this formulation, proven by in vivo experiments on STZ-induced diabetic rats. The suppositories consist of 100 IU/g insulin, 15% poloxamer P407, 20% poloxamer P188, 0.2% polycarbophil, and 10% sodium salicylate. The liquid suppositories were easy to deliver, painless, safe, and remained at administered sites [48,96]. Another mucoadhesive preparation utilises snail mucin motifs extracted from the giant African snail Archachatinamarginata. These mucin motifs (molecular weight 5780Da) contain cysteine residues that form disulphide linkages with cysteine residues in endogenous mucin, leading to sustained release of co-administered insulin. Results indicate that 7% mucin caused 66% reduction of blood glucose levels in rats within 2 hours of administration of the glycerol-gelatin suppository loaded mucin [48,97].
Rectally delivered insulin seems to be a possible alternative for less invasive insulin therapy. Nevertheless, insulin administered through this route appears to have lower bioavailability and efficacy, compared to SC forms, as indicated by experimental results to date. The incorporation of absorption enhancers as well as modification of the suppository formulation to incorporate mucoadhesivity opens up possibilities to improve the delivery of transrectal insulin. However, for long-term therapy, it is worth considering the potential adverse effects, such as increased permeability of rectal mucosa to toxic substances present in the GIT lumen, which may be an unwanted result of using absorption enhancers. Furthermore, rectal delivery is not the most convenient method of administering medications due to the associated discomfort, and suppositories often require special storage conditions. Thus, the improvement in patient compliance from replacing SC insulin with rectal insulin may not be significant [48].
Transdermal insulin: Another method to deliver insulin into the bloodstream in a non-invasive manner is through the skin surface into the underlying capillary network. This method of delivery is convenient, which would lead to better patient compliance. However, the stratum corneum layer on the outermost surface of the skin acts as the main barrier for transdermal delivery, only allowing small lipophilic molecules to be absorbed. Many methods have been developed to overcome this barrier, some of which include chemical absorption enhancers, iontophoresis, sonophoresis/phonophoresis, electroporation, use of microneedles, and laser ablation. Additionally, new drug carriers can also aid transdermal insulin transport (e.g. nano- and microparticles, lipid vesicles) [5,48,146-149].
A study involving insulin-loaded micro-emulsions has shown promising results in achieving transdermal insulin transport through goat skin. Insulin was incorporated into micro-emulsions using oleic acid as the oil phase, Tween 80 as the surfactant, and isopropyl alcohol as the co-surfactant. It was found that the formulation with 10% oleic acid(38% aqueous phase, 50% surfactant phase) with 2% dimethyl sulfoxide (DMSO) as a permeation enhancer, showed maximum permeation flux through goat skin[98]. Another potential formulation for transdermal insulin delivery is via transferosomal gels containing insulin, which showed transdermal absorption with zero-order kinetics through porcine ear skin, as well as prolonged hypoglycaemic effects (over 24 hours) in diabetic rats [99]. A recent study explored the use of amidated pectin hydrogel matrix patches to deliver insulin to diabetic rats, which proved that this system managed to deliver physiologically relevant amounts of insulin, and that the delivered insulin retained pharmacological activity [100]. Insulin emulgel has also been tested for transdermal delivery. The emulgel was prepared using a combination of carbomer or hydroxypropyl methylcellulose (HPMC) as gelling agents and polysorbate 80 as an emulsifier, with emu oil as a penetration enhancer. Albino rabbits treated with the insulin emulgel alone experienced a drop in blood glucose from initial value of 250(±10) to 185(±7)mg/dl at 120 minutes post-administration. Adding iontophoresis caused a greater hypoglycaemic effect (glucose levels decreased to 125(±5)mg/dl at 120 minutes) [101].
Although a potential non-invasive route for effective insulin therapy, transdermal insulin delivery requires further investigation, including clinical studies, to improve insulin bioavailability, as well as ensuring safety of the drug preparation. This is particularly important for the skin, which is more at risk of adverse immune reactions [48].
Conclusions
These advancements in insulin therapy provide opportunities to overcome the disadvantages of current insulin regimens. Ultra-long acting insulin (e.g. degludec) may lead to a convenient reduction in dose frequency, whereas ultra-short acting forms (e.g. Linjeta™) can avoid hypoglycaemic effects after meals. Artificial pancreas systems resemble physiological insulin, and with improved algorithms, have come ever closer to achieving physiological insulin levels and response to meals, especially when used in combination with new ultra-short acting insulin formulations. Transmucosal routes have shown promising results and may soon allow non-invasive insulin delivery for all diabetic patients. Finally, oral insulin seems to be the ideal formulation, which is being extensively researched by numerous companies and institutions worldwide. Nanotechnology has aided substantially in the improvement of insulin delivery as well.
However, it is wise to be cautious with exploring alternative and novel routes for insulin delivery, as insulin may produce unwanted local effects. It is well known that subcutaneous injections of insulin cause local lipodystrophy, damaging subcutaneous fat tissue and causing a degree of disfigurement [150]. Since insulin is a growth factor, it has the tendency to be mutagenic, which is a concern, as insulin therapy is usually life-long. Such prolonged exposure to a potentially mutagenic drug may adversely affect local tissues [6,10,151]. In addition to adverse effects inherent to insulin, the use of absorption enhancers may present their own problems. The barriers to absorption of drug molecules function to prevent penetration of toxins and pathogenic microorganisms. Disrupting such barriers may not only facilitate insulin absorption, but also transport of unwanted molecules and organisms [152]. In addition to that, hepatic first-pass metabolism may reduce bioavailability of insulin, but this process takes place in normal individuals to reduce hepatic glucose production as well as prevent peripheral hyperinsulinaemic effects [5,6,8-10]. Bypassing the liver to increase insulin bioavailability might cause adverse effects in the long run.
In conclusion, much work needs to be done to achieve a safe, non-invasive, convenient insulin formulation. With the release of Oral-lyn™ and Afrezza®, and preliminary research in countless non-invasive insulin delivery systems, much progress has been made towards achieving this goal. Aided by the advancements in nanomedicine, the vision of an ideal insulin tablet may soon be realised, thus, making insulin injections a thing of the past.
References
-
(2013) Diabetes - Fact Sheet No 312: World Health Organization.
-
Simoni RD, Hill RL, Vaughan M (2002) The Discovery of Insulin: the Work of Frederick Banting and Charles Best. The Journal of Biological Chemistry 277: e15.
-
Frier BM, Fisher M (2006) Diabetes Mellitus. 2006. In: Davidson's Principles and Practice of Medicine [Internet]. Churchill Livingstone.
-
Rosenfeld L (2002) Insulin: discovery and controversy. Clin Chem 48: 2270-2288.
-
Yaturu S (2013) Insulin therapies: Current and future trends at dawn. World J Diabetes 4: 1-7.
-
Chen MC, Sonaje K, Chen KJ, Sung HW (2011) A review of the prospects for polymeric nanoparticle platforms in oral insulin delivery. Biomaterials 32: 9826-9838.
-
Chin RL, Martinez R, Garmel G (1993) Gas gangrene from subcutaneous insulin administration. Am J Emerg Med 11: 622-625.
-
Sonaje K, Lin KJ, Wey SP, Lin CK, Yeh TH, et al. (2010) Biodistribution, pharmacodynamics and pharmacokinetics of insulin analogues in a rat model: Oral delivery using pH-responsive nanoparticles vs. subcutaneous injection. Biomaterials 31: 6849-6858.
-
Arbit E (2004) The physiological rationale for oral insulin administration. Diabetes Technol Ther 6: 510-517.
-
Ahmad A, Othman I, Zaini A, Chowdhury EH (2012) Oral Nano-Insulin Therapy: Current Progress on Nanoparticle-Based Devices for Intestinal Epithelium-Targeted Insulin Delivery. J Nanomedic Nanotechnol: S4-007.
-
Bargman JM (1994) Intraperitoneal versus subcutaneous insulin in patients on nighttime IPD. Adv Perit Dial 10: 116-119.
-
Riddle MC, Bolli GB, Ziemen M, Muehlen-Bartmer I, Bizet F, et al. (2014) New Insulin Glargine 300 Units/mL Versus Glargine 100 Units/mL in People With Type 2 Diabetes Using Basal and Mealtime Insulin: Glucose Control and Hypoglycemia in a 6-Month Randomized Controlled Trial (EDITION 1). Diabetes care 37: 2755-2762.
-
Yki-Jarvinen H, Bergenstal R, Ziemen M, Wardecki M, Muehlen-Bartmer I, et al. (2014) New Insulin Glargine 300 Units/mL Versus Glargine 100 Units/mL in People With Type 2 Diabetes Using Oral Agents and Basal Insulin: Glucose Control and Hypoglycemia in a 6-Month Randomized Controlled Trial (EDITION 2). Diabetes care pii: DC_140990. [Epub ahead of print].
-
Birkeland KI, Home PD, Wendisch U, Ratner RE, Johansen T, et al. (2011) Insulin degludec in type 1 diabetes: a randomized controlled trial of a new-generation ultra-long-acting insulin compared with insulin glargine. Diabetes Care 34: 661-665.
-
Heller S, Buse J, Fisher M, Garg S, Marre M, et al. (2012) Insulin degludec, an ultra-longacting basal insulin, versus insulin glargine in basal-bolus treatment with mealtime insulin aspart in type 1 diabetes (BEGIN Basal-Bolus Type 1): a phase 3, randomised, open-label, treat-to-target non-inferiority trial. Lancet 379: 1489-1497.
-
Rodbard HW, Cariou B, Zinman B, Handelsman Y, Philis-Tsimikas A, et al. (2013) Comparison of insulin degludec with insulin glargine in insulin-naive subjects with Type 2 diabetes: a 2-year randomized, treat-to-target trial. Diabet Med 30: 1298-1304.
-
Ratner RE, Gough SC, Mathieu C, Del Prato S, Bode B, et al. (2013) Hypoglycaemia risk with insulin degludec compared with insulin glargine in type 2 and type 1 diabetes: a pre-planned meta-analysis of phase 3 trials. Diabetes Obes Metab 15: 175-184.
-
Zinman B, Philis-Tsimikas A, Cariou B, Handelsman Y, Rodbard HW, et al. (2012) Insulin degludec versus insulin glargine in insulin-naive patients with type 2 diabetes: a 1-year, randomized, treat-to-target trial (BEGIN Once Long). Diabetes Care 35: 2464-2471.
-
Bode BW, Buse JB, Fisher M, Garg SK, Marre M, et al. (2013) Insulin degludec improves glycaemic control with lower nocturnal hypoglycaemia risk than insulin glargine in basal-bolus treatment with mealtime insulin aspart in Type 1 diabetes (BEGIN((R)) Basal-Bolus Type 1): 2-year results of a randomized clinical trial. Diabet Med 30: 1293-1297.
-
Wakil A, Atkin SL (2012) Efficacy and safety of ultra-long-acting insulin degludec. Ther Adv Endocrinol Metab 3: 55-59.
-
Danne T1, Bolinder J (2011) New insulins and insulin therapy. Int J Clin Pract: 26-30.
-
Rodbard HW, Cariou B, Zinman B, Handelsman Y, Wolden ML, et al. (2014) Health status and hypoglycaemia with insulin degludec versus insulin glargine: a 2-year trial in insulin-naïve patients with type 2 diabetes. Diabetes Obes Metab 16: 869-872
-
Zinman B, Fulcher G, Rao PV, Thomas N, Endahl LA, et al. (2011) Insulin degludec, an ultra-long-acting basal insulin, once a day or three times a week versus insulin glargine once a day in patients with type 2 diabetes: a 16-week, randomised, open-label, phase 2 trial. Lancet 377: 924-931.
-
Zinman B, DeVries JH, Bode B, Russell-Jones D, Leiter LA, et al. (2013) Efficacy and safety of insulin degludec three times a week versus insulin glargine once a day in insulin-naive patients with type 2 diabetes: results of two phase 3, 26 week, randomised, open-label, treat-to-target, non-inferiority trials. The Lancet Diabetes & Endocrinology 1: 123-131.
-
Koehler G, Heller S, Korsatko S, Roepstorff C, Rasmussen S, et al. (2014) Insulin degludec is not associated with a delayed or diminished response to hypoglycaemia compared with insulin glargine in type 1 diabetes: a double-blind randomised crossover study. Diabetologia 57: 40-49.
-
Hirsch IB, Bode B, Courreges JP, Dykiel P, Franek E, et al. (2012) Insulin degludec/insulin aspart administered once daily at any meal, with insulin aspart at other meals versus a standard basal-bolus regimen in patients with type 1 diabetes: a 26-week, phase 3, randomized, open-label, treat-to-target trial. Diabetes Care 35: 2174-2181.
-
Schmidt TA, Rosen CJ, Yudkin JS (2013) European Medicines Agency must take account of cardiovascular harm associated with degludec insulin. BMJ 346: f3731.
-
Tucker ME (2013) FDA Rejects Novo Nordisk's Insulin Degludec: Medscape. [http://www.medscape.com/viewarticle/779077]
-
Aye MM, Atkin SL2 (2014) Patient safety and minimizing risk with insulin administration - role of insulin degludec. Drug Healthc Patient Saf 6: 55-67.
-
Berard L, MacNeill G2 (2014) Insulin Degludec, a Long-Acting Once-Daily Basal Analogue for Type 1 and Type 2 Diabetes Mellitus. Can J Diabetes.
-
Davies MJ, Gross JL, Ono Y, Sasaki T, Bantwal G, et al. (2014) Efficacy and safety of insulin degludec given as part of basal-bolus treatment with mealtime insulin aspart in type 1 diabetes: a 26-week randomized, open-label, treat-to-target non-inferiority trial. Diabetes Obes Metab 16: 922-930.
-
Steiner S, Hompesch M, Pohl R, Simms P, Flacke F, et al. (2008) A novel insulin formulation with a more rapid onset of action. Diabetologia 51: 1602-1606.
-
Heinemann L, Nosek L, Flacke F, Albus K, Krasner A, et al. (2012) U-100, pH-Neutral formulation of VIAject(®) : faster onset of action than insulin lispro in patients with type 1 diabetes. Diabetes Obes Metab 14: 222-227.
-
Hompesch M, McManus L, Pohl R, Simms P, Pfutzner A, et al. (2008) Intra-individual variability of the metabolic effect of a novel rapid-acting insulin (VIAject) in comparison to regular human insulin. J Diabetes Sci Technol 2: 568-571.
-
Forst T, Pfützner A, Flacke F, Krasner A, Hohberg C, et al. (2010) Postprandial vascular effects of VIAject compared with insulin lispro and regular human insulin in patients with type 2 diabetes. Diabetes Care 33: 116-120.
-
Heinemann L, Hompesch M, Flacke F, Simms P, Pohl R, et al. (2011) Reduction of postprandial glycemic excursions in patients with type 1 diabetes: a novel human insulin formulation versus a rapid-acting insulin analog and regular human insulin. J Diabetes Sci Technol 5: 681-686.
-
Cobelli C, Renard E, Kovatchev B (2011) Artificial pancreas: past, present, future. Diabetes 60: 2672-2682.
-
Del Favero S, Bruttomesso D, Di Palma F, Lanzola G, Visentin R, et al. (2014) First use of model predictive control in outpatient wearable artificial pancreas. Diabetes Care 37: 1212-1215.
-
Nimri R, Phillip M (2014) Artificial pancreas: fuzzy logic and control of glycemia. Curr Opin Endocrinol Diabetes Obes 21: 251-256.
-
Song L, Zhi ZL, Pickup JC (2014) Nanolayer encapsulation of insulin-chitosan complexes improves efficiency of oral insulin delivery. Int J Nanomedicine 9: 2127-2136.
-
Siddhartha TV, Senthil V, Kishan IS, Khatwal RB, Madhunapantula SV1 (2014) Design and development of oral nanoparticulated insulin in multiple emulsion. Curr Drug Deliv 11: 472-485..
-
Tahtat D, Mahlous M, Benamer S, Khodja AN, Oussedik-Oumehdi H, et al. (2013) Oral delivery of insulin from alginate/chitosan crosslinked by glutaraldehyde. Int J Biol Macromol 58: 160-168.
-
Khedkar A, Iyer H, Anand A, Verma M, Krishnamurthy S, et al. (2010) A dose range finding study of novel oral insulin (IN-105) under fed conditions in type 2 diabetes mellitus subjects. Diabetes Obes Metab 12: 659-664.
-
Heinemann L, Jacques Y (2009) Oral insulin and buccal insulin: a critical reappraisal. J Diabetes Sci Technol 3: 568-584.
-
Pozzilli P, Raskin P, Parkin CG (2010) Review of clinical trials: update on oral insulin spray formulation. Diabetes Obes Metab 12: 91-96.
-
Morales JO, Huang S2, Williams RO 3rd2, McConville JT3 (2014) Films loaded with insulin-coated nanoparticles (ICNP) as potential platforms for peptide buccal delivery. Colloids Surf B Biointerfaces 122: 38-45.
-
Giovino C, Ayensu I, Tetteh J, Boateng JS (2013) An integrated buccal delivery system combining chitosan films impregnated with peptide loaded PEG-b-PLA nanoparticles. Colloids Surf B Biointerfaces 112: 9-15.
-
Khafagy el-S, Morishita M, Onuki Y, Takayama K (2007) Current challenges in non-invasive insulin delivery systems: a comparative review. Adv Drug Deliv Rev 59: 1521-1546.
-
Venugopalan P, Sapre A, Venkatesan N, Vyas SP (2001) Pelleted bioadhesive polymeric nanoparticles for buccal delivery of insulin: preparation and characterization. Pharmazie 56: 217-219.
-
Yang TZ, Wang XT, Yan XY, Zhang Q (2002) Phospholipid deformable vesicles for buccal delivery of insulin. Chem Pharm Bull (Tokyo) 50: 749-753.
-
Xu HB, Huang KX, Zhu YS, Gao QH, Wu QZ, et al. (2002) Hypoglycaemic effect of a novel insulin buccal formulation on rabbits. Pharmacol Res 46: 459-467.
-
Morishita M, Barichello JM, Takayama K, Chiba Y, Tokiwa S, et al. (2001) Pluronic F-127 gels incorporating highly purified unsaturated fatty acids for buccal delivery of insulin. Int J Pharm 212: 289-293.
-
StarokadomskiĬ PL, DubeĬ IIa (2005) [Lysalbinic acid--a new absorption enhancer for the buccal delivery of peptide drugs]. Ukr Biokhim Zh 77: 79-85.
-
Starokadomskyy PL, Dubey IY (2006) New absorption promoter for the buccal delivery: preparation and characterization of lysalbinic acid. Int J Pharm 308: 149-154.
-
FDA approves Afrezza to treat diabetes.
-
Lee HK, Kwon JH, Park SH, Kim CW (2006) Enhanced hypoglycemic activity following intratracheal administration of insulin microcrystal suspension with injection adjuvant. Biosci Biotechnol Biochem 70: 1003-1005.
-
Kwon JH, Lee BH, Lee JJ, Kim CW (2004) Insulin microcrystal suspension as a long-acting formulation for pulmonary delivery. European journal of pharmaceutical sciences: official journal of the European Federation for Pharmaceutical Sciences 22: 107-116.
-
Surendrakumar K, Martyn GP, Hodgers EC, Jansen M, Blair JA (2003) Sustained release of insulin from sodium hyaluronate based dry powder formulations after pulmonary delivery to beagle dogs. J Control Release 91: 385-394.
-
Mitra R, Pezron I, Li Y, Mitra AK (2001) Enhanced pulmonary delivery of insulin by lung lavage fluid and phospholipids. Int J Pharm 217: 25-31.
-
Todo H, Okamoto H, Iida K, Danjo K (2001) Effect of additives on insulin absorption from intratracheally administered dry powders in rats. Int J Pharm 220: 101-110.
-
Hussain A, Yang T, Zaghloul AA, Ahsan F (2003) Pulmonary absorption of insulin mediated by tetradecyl-beta-maltoside and dimethyl-beta-cyclodextrin. Pharm Res 20: 1551-1557.
-
Suarez S, Garcia-Contreras L, Sarubbi D, Flanders E, O'Toole D, et al. (2001) Facilitation of pulmonary insulin absorption by H-MAP: pharmacokinetics and pharmacodynamics in rats. Pharm Res 18: 1677-1684.
-
Huang YY, Wang CH (2006) Pulmonary delivery of insulin by liposomal carriers. J Control Release 113: 9-14.
-
Garcia-Contreras L, Morçöl T, Bell SJ, Hickey AJ (2003) Evaluation of novel particles as pulmonary delivery systems for insulin in rats. AAPS PharmSci 5: E9.
-
Kawashima Y, Yamamoto H, Takeuchi H, Fujioka S, Hino T (1999) Pulmonary delivery of insulin with nebulized DL-lactide/glycolide copolymer (PLGA) nanospheres to prolong hypoglycemic effect. J Control 62: 279-287.
-
Zhang Q, Shen Z, Nagai T (2001) Prolonged hypoglycemic effect of insulin-loaded polybutylcyanoacrylate nanoparticles after pulmonary administration to normal rats. I J Pharm 218: 75-80.
-
Pillion DJ, Ahsan F, Arnold JJ, Balusubramanian BM, Piraner O, et al. (2002) Synthetic long-chain alkyl maltosides and alkyl sucrose esters as enhancers of nasal insulin absorption. J Pharm Sci 91: 1456-1462.
-
Pillion DJ, Atchison JA, Gargiulo C, Wang RX, Wang P, et al. (1994) Insulin delivery in nosedrops: new formulations containing alkylglycosides. Endocrinology 135: 2386-2391.
-
Ahsan F, Arnold JJ, Meezan E, Pillion DJ (2003) Sucrose cocoate, a component of cosmetic preparations, enhances nasal and ocular peptide absorption. Int J Pharm 251: 195-203.
-
Najafabadi AR, Moslemi P, Tajerzadeh H (2004) Intranasal bioavailability of insulin from carbopol-based gel spray in rabbits. Drug Deliv 11: 295-300.
-
Varshosaz J, Sadrai H, Heidari A (2006) Nasal delivery of insulin using bioadhesive chitosan gels. Drug Deliv 13: 31-38.
-
Varshosaz J, Sadrai H, Alinagari R (2004) Nasal delivery of insulin using chitosan microspheres. J Microencapsul 21: 761-774.
-
Wang J, Tabata Y, Morimoto K (2006) Aminated gelatin microspheres as a nasal delivery system for peptide drugs: evaluation of in vitro release and in vivo insulin absorption in rats. J Control Release 113: 31-37.
-
Krauland AH, Leitner VM, Grabovac V, Bernkop-Schnürch A (2006) In vivo evaluation of a nasal insulin delivery system based on thiolated chitosan. J Pharm Sci 95: 2463-2472.
-
Krauland AH, Guggi D, Bernkop-Schnürch A (2006) Thiolated chitosan microparticles: a vehicle for nasal peptide drug delivery. Int J Pharm 307: 270-277.
-
Jintapattanakit A, Peungvicha P, Sailasuta A, Kissel T, Junyaprasert VB (2010) Nasal absorption and local tissue reaction of insulin nanocomplexes of trimethyl chitosan derivatives in rats. J Pharm Pharmacol 62: 583-591.
-
Simon M, Wittmar M, Kissel T, Linn T (2005) Insulin containing nanocomplexes formed by self-assembly from biodegradable amine-modified poly(vinyl alcohol)-graft-poly(L-lactide): bioavailability and nasal tolerability in rats. Pharm Res 22: 1879-1886.
-
Zheng C, Guo Q, Wu Z, Sun L, Zhang Z, et al. (2013) Amphiphilic glycopolymer nanoparticles as vehicles for nasal delivery of peptides and proteins. European Journal of Pharmaceutical Sciences 49: 474-482.
-
Yamamoto A, Luo AM, Dodda-Kashi S, Lee VH (1989) The ocular route for systemic insulin delivery in the albino rabbit. J Pharmacol Exp Ther 249: 249-255.
-
Pillion DJ, Atchison JA, Wang RX, Meezan E (1994) Alkylglycosides enhance systemic absorption of insulin applied topically to the rat eye. J Pharmacol Exp Ther 271: 1274-1280.
-
Xuan B, McClellan DA, Moore R, Chiou GC (2005) Alternative delivery of insulin via eye drops. Diabetes Technol Ther 7: 695-698.
-
Morgan RV (1995) Delivery of systemic regular insulin via the ocular route in cats. J Ocul Pharmacol Ther 11: 565-573.
-
Morgan RV, Huntzicker MA (1996) Delivery of systemic regular insulin via the ocular route in dogs. J Ocul Pharmacol Ther 12: 515-526.
-
Srinivasan R, Jain SK (1998) Insulin delivery through the ocular route. Drug Deliv 5: 53-55.
-
Lee YC, Simamora P, Yalkowsky SH (1997) Effect of Brij-78 on systemic delivery of insulin from an ocular device. J Pharm Sci 86: 430-433.
-
Lee YC, Yalkowsky SH (1999) Ocular devices for the controlled systemic delivery of insulin: in vitro and in vivo dissolution. Int J Pharm 181: 71-77.
-
Lee Y, Yalkowsky SH (1999) Systemic absorption of insulin from a Gelfoam ocular device. Int J Pharm 190: 35-40.
-
Lee YC, Simamora P, Yalkowsky SH (1997) Systemic delivery of insulin via an enhancer-free ocular device. J Pharm Sci 86: 1361-1364.
-
Lee YC, Yalkowsky SH (1999) Effect of formulation on the systemic absorption of insulin from enhancer-free ocular devices. Int J Pharm 185: 199-204.
-
Hosny EA (1999) Relative hypoglycemia of rectal insulin suppositories containing deoxycholic acid, sodium taurocholate, polycarbophil, and their combinations in diabetic rabbits. Drug Dev Ind Pharm 25: 745-752.
-
Hosny EA, Al-Shora HI, Elmazar MM (2001) Relative hypoglycemic effect of insulin suppositories in diabetic beagle dogs: optimization of various concentrations of sodium salicylate and polyoxyethylene-9-lauryl ether. Biol Pharm Bull 24: 1294-1297.
-
Hosny EA, Al-Shora HI, Elmazar MM (2001) Effect of different bile salts on the relative hypoglycemia of witepsol W35 suppositories containing insulin in diabetic Beagle dogs. Drug Dev Ind Pharm 27: 837-845.
-
Değim Z, Değim T, Acartürk F, Erdoğan D, Ozoğul C, et al. (2005) Rectal and vaginal administration of insulin-chitosan formulations: an experimental study in rabbits. J Drug Target 13: 563-572.
-
Hosny E, el-Ahmady O, el-Shattawy H, Nabih A el-M, el-Damacy H, et al. (1994) Effect of sodium salicylate on insulin rectal absorption in humans. Arzneimittelforschung 44: 611-613.
-
Hosny EA, Al-Marzouki ZM, Metwally ME, Souaida MY, Alshaik AR (2003) Evaluation of efficiency of insulin suppository formulations containing sodium salicylate or sodium cholate in insulin dependent diabetic patients. Boll Chim Farm 142: 361-366.
-
Yun M, Choi H, Jung J, Kim C (1999) Development of a thermo-reversible insulin liquid suppository with bioavailability enhancement. Int J Pharm 189: 137-145.
-
Adikwu MU (2005) Evaluation of snail mucin motifs as rectal absorption enhancer for insulin in non-diabetic rat models. Biol Pharm Bull 28: 1801-1804.
-
Malakar J, Sen SO, Nayak AK, Sen KK (2011) Development and evaluation of microemulsions for transdermal delivery of insulin. ISRN Pharm 2011: 780150.
-
Malakar J, Sen SO, Nayak AK, Sen KK (2012) Formulation, optimization and evaluation of transferosomal gel for transdermal insulin delivery. Saudi Pharm J 20: 355-363.
-
Hadebe SI, Ngubane PS, Serumula MR, Musabayane CT (2014) Transdermal delivery of insulin by amidated pectin hydrogel matrix patch in streptozotocin-induced diabetic rats: effects on some selected metabolic parameters. PloS one 9: e101461.
-
Akram M, Naqvi SB, Khan A (2013) Design and development of insulin emulgel formulation for transdermal drug delivery and its evaluation. Pak J Pharm Sci 26: 323-332.
-
Breton M, Farret A, Bruttomesso D, Anderson S, Magni L, et al. (2012) Fully integrated artificial pancreas in type 1 diabetes: modular closed-loop glucose control maintains near normoglycemia. Diabetes 61: 2230-2237.
-
Guerra S, Facchinetti A, Sparacino G, Nicolao GD, Cobelli C (2012) Enhancing the accuracy of subcutaneous glucose sensors: a real-time deconvolution-based approach. IEEE Trans Biomed Eng 59: 1658-1669.
-
Hompesch M, Muchmore DB, Morrow L, Vaughn DE (2011) Accelerated insulin pharmacokinetics and improved postprandial glycemic control in patients with type 1 diabetes after coadministration of prandial insulins with hyaluronidase. Diabetes care 34: 666-668.
-
Hompesch M, Muchmore DB, Morrow L, Ludington E, Vaughn DE (2012) Improved postprandial glycemic control in patients with type 2 diabetes from subcutaneous injection of insulin lispro with hyaluronidase. Diabetes Technol Ther 14: 218-224.
-
Kovatchev BP, Renard E, Cobelli C, Zisser HC, Keith-Hynes P, et al. (2013) Feasibility of outpatient fully integrated closed-loop control: first studies of wearable artificial pancreas. Diabetes Care 36: 1851-1858.
-
Kovatchev BP, Renard E2, Cobelli C3, Zisser HC4, Keith-Hynes P5, et al. (2014) Safety of outpatient closed-loop control: first randomized crossover trials of a wearable artificial pancreas. Diabetes Care 37: 1789-1796.
-
Galloway JA, Chance RE (1994) Improving insulin therapy: achievements and challenges. Horm Metab Res 26: 591-598.
-
Sood A, Panchagnula R (2001) Peroral route: an opportunity for protein and peptide drug delivery. Chem Rev 101: 3275-3303.
-
Bernkop-Schnürch A (1998) The use of inhibitory agents to overcome the enzymatic barrier to perorally administered therapeutic peptides and proteins. J Control Release 52: 1-16.
-
Chang LL, Bai JP (1996) Evidence for the existence of insulin-degrading enzyme on the brush-border membranes of rat enterocytes. Pharm Res 13: 801-803.
-
Langguth P, Bohner V, Heizmann J, Merkle HP, Wolffram S, et al. (1997) The challenge of proteolytic enzymes in intestinal peptide delivery. Journal of Controlled Release 46: 39-57.
-
Ahmad A, Othman I, Zain AZ, Chowdhury EH1 (2014) Controlled Release of Insulin in Blood Fromstrontium-Substituted Carbonate Apatite Complexes. Curr Drug Deliv.
-
Biocon. Active Development Programs: Biocon.
-
Dave N, Hazra P, Khedkar A, Manjunath HS, Iyer H, et al. (2008) Process and purification for manufacture of a modified insulin intended for oral delivery. J Chromatogr A 1177: 282-286.
-
Sudhakar Y, Kuotsu K, Bandyopadhyay AK (2006) Buccal bioadhesive drug delivery--a promising option for orally less efficient drugs. J Control Release 114: 15-40.
-
Senel S, Hincal AA (2001) Drug permeation enhancement via buccal route: possibilities and limitations. J Control Release 72: 133-144.
-
Tian W, Hu Q, Xu Y, Xu Y (2012) Effect of soybean-lecithin as an enhancer of buccal mucosa absorption of insulin. Biomed Mater Eng 22: 171-178.
-
Senel S, Kremer M, Nagy K, Squier C (2001) Delivery of bioactive peptides and proteins across oral (buccal) mucosa. Curr Pharm Biotechnol 2: 175-186.
-
Agu RU, Ugwoke MI, Armand M, Kinget R, Verbeke N (2001) The lung as a route for systemic delivery of therapeutic proteins and peptides. Respir Res 2: 198-209.
-
DeFronzo RA, Bergenstal RM, Cefalu WT, Pullman J, Lerman S, et al. (2005) Efficacy of inhaled insulin in patients with type 2 diabetes not controlled with diet and exercise: a 12-week, randomized, comparative trial. Diabetes Care 28: 1922-1928.
-
Rosenstock J, Zinman B, Murphy LJ, Clement SC, Moore P, et al. (2005) Inhaled insulin improves glycemic control when substituted for or added to oral combination therapy in type 2 diabetes: a randomized, controlled trial. Ann Intern Med 143: 549-558.
-
Skyler JS, Weinstock RS, Raskin P, Yale JF, Barrett E, et al. (2005) Use of inhaled insulin in a basal/bolus insulin regimen in type 1 diabetic subjects: a 6-month, randomized, comparative trial. Diabetes Care 28: 1630-1635.
-
Himmelmann A, Jendle J, Mellén A, Petersen AH, Dahl UL, et al. (2003) The impact of smoking on inhaled insulin. Diabetes Care 26: 677-682.
-
Henry RR1, Mudaliar SR, Howland WC 3rd, Chu N, Kim D, et al. (2003) Inhaled insulin using the AERx Insulin Diabetes Management System in healthy and asthmatic subjects. Diabetes Care 26: 764-769.
-
Kim D, Mudaliar S, Chinnapongse S, Chu N, Boies SM, et al. (2003) Dose-response relationships of inhaled insulin delivered via the Aerodose insulin inhaler and subcutaneously injected insulin in patients with type 2 diabetes. Diabetes care 26: 2842-2847.
-
Gale EA (2001) Two cheers for inhaled insulin. Lancet 357: 324-325.
-
Stoever JA, Palmer JP (2002) Inhaled insulin and insulin antibodies: a new twist to an old debate. Diabetes Technol Ther 4: 157-161.
-
Gerber RA, Cappelleri JC, Kourides IA, Gelfand RA (2001) Treatment satisfaction with inhaled insulin in patients with type 1 diabetes: a randomized controlled trial. Diabetes Care 24: 1556-1559.
-
Hinchcliffe M, Illum L (1999) Intranasal insulin delivery and therapy. Adv Drug Deliv Rev 35: 199-234.
-
Türker S, Onur E, Ozer Y (2004) Nasal route and drug delivery systems. Pharm World Sci 26: 137-142.
-
Pringels E, Callens C, Vervaet C, Dumont F, Slegers G, et al. (2006) Influence of deposition and spray pattern of nasal powders on insulin bioavailability. Int J Pharm 310: 1-7.
-
Soane RJ, Frier M, Perkins AC, Jones NS, Davis SS, et al. (1999) Evaluation of the clearance characteristics of bioadhesive systems in humans. Int J Pharm 178: 55-65.
-
Soane RJ, Hinchcliffe M, Davis SS, Illum L (2001) Clearance characteristics of chitosan based formulations in the sheep nasal cavity. Int J Pharm 217: 183-191.
-
Illum L (1998) Chitosan and its use as a pharmaceutical excipient. Pharm Res 15: 1326-1331.
-
Dyer AM, Hinchcliffe M, Watts P, Castile J, Jabbal-Gill I, et al. (2002) Nasal delivery of insulin using novel chitosan based formulations: a comparative study in two animal models between simple chitosan formulations and chitosan nanoparticles. Pharm Res 19: 998-1008.
-
Wheatley M, Dent J, Wheeldon E, Smith P (1988) Nasal drug delivery: An in vitro characterization of transepithelial electrical properties and fluxes in the presence or absence of enhancers. Journal of Controlled Release 8: 167-177.
-
Shao Z, Mitra AK (1992) Nasal membrane and intracellular protein and enzyme release by bile salts and bile salt-fatty acid mixed micelles: correlation with facilitated drug transport. Pharm Res 9: 1184-1189.
-
Schipper N, Verhoef J, Romeijn S, Merkus F (1992) Absorption enhancers in nasal insulin delivery and their influence on nasal ciliary functioning. Journal of Controlled Release 21: 173-185.
-
Chiou GC (1994) Systemic delivery of polypeptide drugs through ocular route. J Ocul Pharmacol 10: 93-99.
-
Bartlett JD, Turner-Henson A, Atchison JA, Woolley TW, Pillion DJ (1994) Insulin administration to the eyes of normoglycemic human volunteers. J Ocul Pharmacol 10: 683-690.
-
Pillion DJ, Atchison JA, Stott J, McCracken D, Gargiulo C, et al. (1994) Efficacy of insulin eyedrops. J Ocul Pharmacol 10: 461-470.
-
Soni V, Singh R, Srinivasan R, Jain SK (1998) Pulsatile insulin delivery through the ocular route. Drug Deliv 5: 47-51.
-
Chiou GC, Li BH (1993) Chronic systemic delivery of insulin through the ocular route. J Ocul Pharmacol 9: 85-90.
-
Rocha EM, Cunha DA, Carneiro EM, Boschero AC, Saad MJ, et al. (2002) Identification of insulin in the tear film and insulin receptor and IGF-1 receptor on the human ocular surface. Invest Ophthalmol Vis Sci 43: 963-967.
-
Williams AC, Barry BW (2004) Penetration enhancers. Adv Drug Deliv Rev 56: 603-618.
-
Kalia YN, Naik A, Garrison J, Guy RH (2004) Iontophoretic drug delivery. Adv Drug Deliv Rev 56: 619-658.
-
Lavon I, Kost J (2004) Ultrasound and transdermal drug delivery. Drug Discov Today 9: 670-676.
-
Prausnitz MR (2004) Microneedles for transdermal drug delivery. Adv Drug Deliv Rev 56: 581-587.
-
Hajheydari Z, Kashi Z, Akha O, Akbarzadeh S (2011) Frequency of lipodystrophy induced by recombinant human insulin. Eur Rev Med Pharmacol Sci 15: 1196-1201.
-
Brogden RN, Heel RC (1987) Human insulin. A review of its biological activity, pharmacokinetics and therapeutic use. Drugs 34: 350-371.
-
Sousa S, Lecuit M, Cossart P (2005) Microbial strategies to target, cross or disrupt epithelia. Curr Opin Cell Biol 17: 489-498.
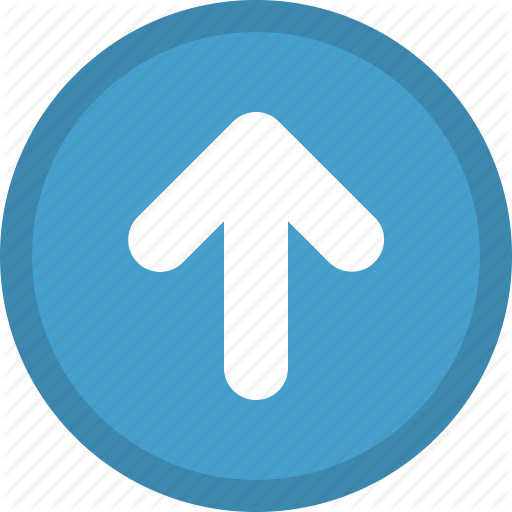