International Journal of Radiology and Imaging Technology
Does Improved Technology in SPECT Myocardial Perfusion Imaging Reduce Downstream Costs? An Observational Study
Nicholas D Patchett1, Sumeet Pawar1, Aaron Sverdlov2 and Edward J Miller3*
1Department of Internal Medicine, Boston University School of Medicine, USA
2Department of Cardiology, University of Adelaide, Australia
3Department of Internal Medicine, Yale University School of Medicine, USA
*Corresponding author:
Edward J Miller, Assistant Professor of Medicine and Radiology, Department of Internal Medicine, Yale University School of Medicine, Section of Cardiovascular Medicine, 333 Cedar Street, New Haven, CT 06520, USA, Tel: 203-785-4129, Fax: 203-785-7144, E-mail: edward.miller@yale.edu
Int J Radiol Imaging Technol, IJRIT-3-023, (Volume 3, Issue 1), Original Research
Received: October 25, 2016 | Accepted: February 22, 2017 | Published: February 25, 2017
Citation: Patchett ND, Pawar S, Sverdlov A, Miller EJ (2017) Does Improved Technology in SPECT Myocardial Perfusion Imaging Reduce Downstream Costs? An Observational Study. Int J Radiol Imaging Technol 3:023.
Copyright: © 2017 Patchett ND, et al. This is an open-access article distributed under the terms of the Creative Commons Attribution License, which permits unrestricted use, distribution, and reproduction in any medium, provided the original author and source are credited.
Abstract
Background: Advances in myocardial perfusion imaging (MPI), including CT attenuation correction (CTAC) and advanced image reconstruction algorithms, result in improved image quality, thus reducing equivocal or false positive results. This theoretically decreases the need for downstream testing; however, there is limited access to these technologies due to their costs and the magnitude of benefit remains unclear. We performed an observational study to evaluate the effect of implementing a SPECT/CT system with advanced image reconstruction algorithms on diagnostic accuracy of MPI, and its effect on downstream healthcare expenditures.
Methods: 277 consecutive patients who underwent SPECT MPI with a traditional SPECT camera were compared to 273 patients imaged at the same institution with a new SPECT/CT system with advanced image reconstruction algorithms. Accuracy of MPI was assessed in a subgroup of 36 and 26 consecutive patients in the traditional SPECT and SPECT/CT groups respectively, who underwent clinically-indicated invasive coronary angiography (ICA).
Utilization of medical services related to coronary artery disease (CAD) was tabulated for the year following SPECT MPI and mean cost of services was estimated using national Medicare reimbursement data.
Results: MPI studies performed on the SPECT/CT system were more likely to be overall true positive (62% vs. 25%; p = 0.008) and less likely to be false positive (19% vs. 56%; p = 0.004). Fewer false positive MPI studies resulted in fewer patients undergoing diagnostic ICA procedures (4% vs. 9%; p = 0.03). Among patients with additional CAD-related services within one year, total downstream healthcare cost was not significantly decreased, but mean expenditure on diagnostic healthcare services was lower in the SPECT/CT group ($4288 vs. $5740; p = 0.04).
Conclusions: Use of SPECT/CT with advanced image reconstruction software for MPI can result in more efficient downstream utilization of diagnostic ICA and reduced downstream diagnostic expenditures.
Keywords
Myocardial perfusion imaging, SPECT, SPECT/CT, Resolution recovery, Healthcare costs
Introduction
Myocardial perfusion imaging (MPI) plays a key role in the diagnosis, establishing prognosis, and assessing the effectiveness of therapy in cardiovascular disease, and it has evolved rapidly with improvements in SPECT camera software and hardware [1]. With the development of hybrid SPECT/CT camera systems, computed tomography-based attenuation correction (CTAC), has become available for routine clinical use. CTAC increases the specificity of SPECT MPI for detection of significant coronary lesions [2-4], but with variable effects on sensitivity [5-8] and on clinical outcomes [9,10]. Additionally, SPECT image reconstruction software can include advanced algorithms for resolution recovery (RR) and scatter correction (SC), which improve the signal-to-noise ratio of SPECT images, enabling use of lower radiotracer doses without loss of diagnostic accuracy [11-13] or a worsened clinical outcome [14]. Finally, new cardiac-only SPECT systems using solid-state detectors are now broadly available, which improve diagnostic accuracy, as well as decrease image acquisition time and patient radiation exposure [15-18].
Despite these technological advancements, many imaging centers continue to perform MPI using older general-purpose SPECT systems without these advances. While a cardiac-only SPECT system may only be practical at centers where the volume of MPI studies is large enough to drive full utilization of a dedicated cardiac SPECT camera, upgrading to a newer general-purpose, wide field-of-view SPECT/CT system would be potentially feasible at nuclear medicine practices of any size, albeit at a higher cost. Unfortunately, we couldn't find any data available on the downstream effects of SPECT/CT and advanced image processing algorithms on quality of care and healthcare expenditures, on our careful literature review. Therefore, the material value of upgrading older equipment remains unclear.
Our academic nuclear cardiology laboratory recently replaced an older SPECT camera with line-source attenuation correction (Siemanse CAM - Siemens AG: Munich, Germany) with a new SPECT/CT system with image reconstruction software able to perform RR and SC (GE Discovery NM/CT 670 SPECT/CT with GE Evolution® software - General Electric: Fairfield, Connecticut). Both the old SPECT and new SPECT/CT cameras are general-purpose instruments utilizing traditional photomultiplier tube technology. The objective of this study was to assess the impact this change had on quality and cost of patient care. We hypothesized that the new SPECT/CT system would have improved diagnostic accuracy, resulting in a decrease in downstream diagnostic healthcare services and a reduction in the associated preventable healthcare costs.
Methods
Patient cohorts
The Boston University Medical Center IRB approved this study and granted waiver of informed consent. Two cohorts of patients were identified using an automated query of our hospital's stress-testing database. The "SPECT group" included all patients who underwent MPI during the final four months of use of a Siemens eCAM with Gd-153 line-source attenuation correction. The "SPECT/CT" group included all patients who underwent MPI during a five-month period starting one year after replacement of the Siemens eCAM with a new GE Discovery NM/CT670. We elected not to include the first year of data to allow for protocol optimization and experience with the new equipment. Duration of the two cohorts was set such that each contained at least 250 consecutive patients, which we estimated was the approximate sample size required to demonstrate a 20% difference in preventable diagnostic expenditures at a power of 0.8. Retrospective review of electronic medical records was performed to collect relevant clinical data on all study patients.
Camera systems and image acquisition protocols
SPECT group: MPI was performed on a Siemens eCAM with Gd-153 line-source attenuation correction (AC). ECG-gated SPECT images were acquired using weight-based Tc-99 m sestamibi dosing (< 200 lbs: 10 mCi rest, 30 mCi stress; 200-250 lbs: 12 mCi rest, 36 mCi stress; > 250 lbs: 45 mCi stress, 45 mCi rest). Acquisition parameters included: 90° dual-head configuration with 180° orbit (32 intervals, 25 seconds/step), LEHR collimator, 20% energy window centered at 140 keV, 128×128 matrix. Non-AC images were processed using filtered back protection (FBP) with a Butterworth filter (order 5, cut-off 0.35); AC images were processed by ordered subset optimization maximization (OSEM) (4 iterations, 12 subsets).
SPECT/CT group: MPI was performed on a GE NM 670 system with 16-slice CT scanner for CTAC (GE Bright Speed Elite). ECG-gated SPECT images were acquired using BMI-based Tc-99 m sestamibi dosing (BMI < 20: 6 mCi rest, 20 mCi stress; BMI 21-30: 8 mCi rest, 25 mCi stress; BMI 31-35: 10 mCi rest, 30 mCi stress; BMI > 35: 30 mCi stress, 30 mCi rest). Acquisition parameters included: 90° dual-head configuration with a 180° orbit (32 intervals, 20 seconds per step), LEHR collimator, 20% energy window centered at 140 keV, 64×64 matrix. CT parameters included: 1.0 sec rotation, pitch 0.875:1, 10 mA, 120 kV, 512×512 matrix. Non-AC images were processed by FBP with a Butterworth filter (order 105, cut-off 0.435). AC images were processed by OSEM (12 iterations, 10 subsets) with RR and SC (GE Evolution®).
The scan time was weight-based (BMI < 35 = 10.7 min, BMI > 35 = 13.3 min). Each study was clinically interpreted by one of four experienced readers. Clinical interpretation from the study report was used for imaging findings. Interpreting physicians and image interpretation software (Invia 4DM - Invia LLC: Ann Arbor Michigan) were unchanged between the two cohorts.
Accuracy of MPI versus invasive coronary angiography
Diagnostic accuracy of MPI was assessed against invasive coronary angiography (ICA) in the subgroup of consecutive patients from each cohort who underwent clinically-indicated ICA. To increase the probability of identifying any patients with false negative MPI studies, ICA procedures performed up to one year after MPI were used for this analysis. Locations of hemodynamically significant stenoses were identified by review of clinical ICA reports. Lesions were considered obstructive based on the visual assessment of the operator (left main > 50%; LAD, LCx, RCA > 70%). Locations of stenoses were then compared to locations of defects on MPI by review of clinical MPI reports. The vascular distribution associated with any observed perfusion defects was assigned according a standard 17-segment model [19] for patients with typical coronary anatomy, and was assessed on a case-by-case basis for those with variant anatomy.
Each MPI result was judged as true positive (TP), false positive (FP), true negative (TN), or false negative (FN) in the distribution of each of the three coronary arteries, and for the test overall. Abnormal MPI studies were classified as FP overall if any vascular distribution was a false positive. They were described as TP overall if at least one vascular distribution was a true positive result and no other vascular distributions were false positives. Normal MPI studies were classified as TN or FN overall based on presence or absence of obstructive coronary artery disease (CAD) on subsequent ICA. Frequencies of TP, FP, TN, and FN results were compared between the SPECT and SPECT/CT groups. Because the frequencies of the four test outcomes in any given vascular distribution are closely related observations, a Bonferroni-corrected value of 0.0125 (0.05 ÷ 4) was used to define a significant p-value.
Positive predictive value (PPV) and sensitivity (Sn) were calculated for each vessel and for the study as a whole. Given the retrospective study design, we assumed there would be a significant bias against performing ICA on patients with normal MPI; because this would artificially decrease the number of confirmed TN and FN studies we elected not to calculate the test's specificity and negative predictive value. To contextualize any observed differences in diagnostic accuracy, average patient radiation exposure was calculated for each group (in the SPECT/CT cohort the dose-length product for the CT was included in the total).
Downstream healthcare utilization and expenditures
Downstream utilization of medical services related to the diagnosis or treatment of CAD was tabulated and compared for a one-year period following SPECT MPI. Total expenditure on CAD-related healthcare services within one year was estimated, as was the total expenditure on purely diagnostic services that were potentially preventable (repeat stress tests, admissions for chest pain with negative cardiac enzymes, and diagnostic ICA procedures where in revascularization was neither performed nor planned for a future time). Cost of each hospital admission was estimated using national average Medicare reimbursements [20] and cost of each outpatient procedure or test was estimated using the maximum allowed Medicare reimbursement for that procedure [21]. To clinically contextualize the estimates of healthcare expenditure, incidence of myocardial infarction and all-cause mortality was also recorded for each cohort.
Statistical analysis
Statistical analysis was performed in SPSS (version 22, IBM). Unpaired two-tailed t-test was used for comparison of continuous variables, Mann-Whitney U for ordinal variables, and chi-squared test or Fisher's exact test as appropriate for categorical variables. Significance was assessed at α = 0.05 unless otherwise stated, with Bonferroni correction used wherever a group of comparisons were closely related. The 95% confidence interval (CI) of diagnostic statistics values was estimated using the Wald method [22].
Binary logistic backward regression analysis was performed on the subgroup of patients who underwent ICA to assess for confounding variables predictive of TP MPI results. Variables were included in a univariate screen if they could be expected to correlate with presence of CAD (hyperlipidemia, diabetes, hypertension, age, sex, prior revascularization, LVEF < 50%) or accuracy of SPECT imaging (SPECT versus SPECT/CT, sum difference score, body mass index, pharmacologic versus exercise stress). Covariates were selected for multivariate analysis if the univariate odds ratio for TP test outcome was significant at α < 0.2.
Results
Baseline characteristics of study cohorts
277 consecutive patients in the SPECT group were compared to 273 consecutive patients in the SPECT/CT group. Demographic characteristics and baseline risk factors between SPECT and SPECT/CT were generally similar (Table 1). There were slightly fewer patients in the SPECT/CT group with a history of prior coronary artery bypass graft (CABG) (11% versus 6%; p = 0.05), although the percent of patients with prior revascularization was not different overall (21% vs. 18%; p = 0.45). 36 consecutive patients in the SPECT group and 27 consecutive patients in the SPECT/CT group underwent invasive coronary angiography within one year. In this subgroup, the SPECT/CT patients were significantly older (69.7 vs. 63.8 years; p = 0.02).
Table 1: Consecutive patients who underwent traditional SPECT myocardial perfusion imaging (MPI) are compared to consecutive patients who underwent SPECT/CT MPI. The subgroup from each cohort that underwent clinically-indicated invasive coronary angiography (ICA) within one year is also compared. For continuous variables, the standard deviation is included in parentheses.
View Table 1
Besides replacement of the SPECT system, no significant changes were made in our practice between the two temporally consecutive patient cohorts. Rates of SPECT MPI as a percentage of all stress tests performed at our institution did not change between the SPECT and SPECT/CT cohorts(21% vs. 22%; p = 0.35) and the same four nuclear readers interpreted studies during the two time periods, with no significant changes in the distribution of studies between the four providers (p = 0.21). There was no difference in the rate of studies read as overall abnormal (31% vs. 35%; p = 0.36), and the percent of patients undergoing coronary angiography within one year was also not significantly different (13% vs. 10%; p = 0.23).
Accuracy of MPI versus invasive coronary angiography
Diagnostic accuracy of the SPECT and SPECT/CT systems was compared between patients in each cohort who underwent clinically-indicated ICA within one year of their MPI study (Table 2). The prevalence of overall true positive MPI studies was higher in the SPECT/CT group (62% vs. 25%; p = 0.008), while prevalence of overall false positive MPI studies was lower (19% vs. 56%; p = 0.004). These findings correspond to a significant improvement in the PPV of the MPI study overall from 0.28 to 0.76.
Table 2: The prevalence of true positive (TP), false positive (FP), true negative (TN), and false negative (FN) myocardial perfusion imaging (MPI) studies is compared for patients within each cohort that underwent clinically-indicated invasive coronary angiography (ICA) within one year. Positive predictive value (PPV) and sensitivity are calculated for each of the three coronary artery distributions and for the study as a whole.
View Table 2
A trend toward increase in false negative tests was observed in the SPECT/CT group in both RCA (25% vs. 3%; p = 0.02) and LCx distributions (35% vs. 8%; p = 0.02), although the differences did not retain significance after Bonferroni correction. This corresponded to a trend toward decreased sensitivity within both RCA and LCx distributions.
On multivariate analysis (Table 3), use of the SPECT/CT system was found to be a strong independent predictor of true positive MPI results (p = 0.001). The other covariate found to independently predict true positive test outcome was higher SDS (p = 0.04). Although average age differed between the two subgroups of patients who underwent ICA, this variable was not found to be predictive of true positive test results (p = 0.70).
Table 3: The univariate odds ratio that a myocardial perfusion imaging (MPI) study will be true positive is listed for clinically relevant variables. Covariates found to be significant at α < 0.2 were selected for multivariate analysis; multivariate odds ratios are listed at right (with 95% confidence interval and p value).
View Table 3
Average patient radiation exposure (Table 4) was found to have been lower in the SPECT/CT group (11.0 vs. 13.2 mSv; p < 0.001).
Table 4: Mean radiation exposure in millisieverts (mSv) from the index SPECT myocardial perfusion imaging (MPI) study is listed for both cohorts. The total annual exposure to iatrogenic radiation is calculated by including radiation from subsequent MPI studies and invasive coronary angiography (ICA) procedures. Standard deviation is listed in parentheses.
View Table 4
Downstream healthcare utilization and expenditures
In the SPECT/CT group there were several changes in rates of healthcare utilization and downstream healthcare costs during the year following SPECT MPI (Table 5). There was no difference in the number of patients who received at least one additional CAD-related healthcare service (27% vs. 27%; p = 0.99), but there was a significant decrease in the number of patients in the SPECT/CT group who underwent purely diagnostic ICA procedures where no percutaneous intervention (PCI) was performed and no referral was made for CABG (4% vs. 9%; p = 0.03). Mean expenditure on CAD-related healthcare services was estimated for the year following MPI among patients who received any such additional services. National average Medicare reimbursement figures were used (Table 6). The mean overall expenditure on CAD-related services within one year did not decrease at a significant level ($8248 vs. $9001; p = 0.11); however, mean expenditure on purely diagnostic healthcare services was significantly decreased ($4288 versus $5740; p = 0.04).
Table 5: Incidence of medical services related to coronary artery disease (CAD) during the first year following each patient's baseline myocardial perfusion imaging (MPI) study is compared. National Medicare reimbursement data is used to estimate the average total cost per patient. Mean expenditure on preventable diagnostic services is estimated by excluding expenses related to clinically-indicated revascularization procedures.
View Table 5
Table 6: Diagnosis-related group (DRG) codes are listed for all study-relevant inpatient discharge diagnoses; Healthcare Common Procedure Coding System (HCPCS) codes are listed for all relevant outpatient cardiac stress testing. Data is based on 2012 reimbursement figures. All values are listed in US dollars.
View Table 6
No difference was seen in incidence of adverse health outcomes within one year of SPECT MPI. In both the SPECT and SPECT/CT groups, only two deaths occurred. In both groups, 23 patients experienced myocardial infarction (including demand-mediated or "type II" myocardial infarction (MI).
Discussion
Myocardial perfusion imaging (MPI) is a valuable tool in the diagnosis and management of patients with cardiovascular disease. It is also a complex physiologic imaging process, which exposes it to several potential pitfalls and artifacts that can limit the final interpretation of the study. Given recent advancements in SPECT imaging, replacement of any older general-purpose SPECT system that is approaching the end of its service life now requires careful consideration of the financial costs of the equipment versus clinical benefits associated with adopting newer technologies. Investing in a SPECT system with a hybrid CT scanner enables CT-based attenuation correction (CTAC), which is known to improve the specificity of MPI [5-7]. Addition of contemporary image reconstruction software with resolution recovery (RR) and scatter correction (SC) allows for reduction in radiotracer dose without loss of diagnostic accuracy [11,12]. However, adding these technologies to a general-purpose SPECT system can nearly double its cost. To our knowledge, there is no previously published data demonstrating that implementation of these technologies alters healthcare utilization in a way that would economically justify the increased upfront expense. This study demonstrates that replacing an older general-purpose SPECT camera with a general-purpose SPECT/CT system leads to improvement in the PPV of MPI while at the same time reducing patient radiation exposure, and also results in a decrease in the rate of preventable downstream diagnostic invasive coronary angiography (ICA), with an associated decrease in expenditure on preventable healthcare services during the subsequent year. Although this study was not powered for clinical outcomes, these quality improvements and cost savings were achieved without any suggestion of increase in the rate of MI and all-cause mortality.
Our findings on the diagnostic accuracy of our SPECT/CT system are in line with existing knowledge. The SPECT/CT group had fewer false positive (FP) tests, more true positive (TP) results, and a marked improvement in the test's overall PPV. This is consistent with prior data on CTAC, which has demonstrated a decrease in the frequency of false positive MPI studies and a resultant improvement in the test's specificity [5-7].The data suggested an increase in false negative (FN) MPI results for SPECT/CT in the RCA and LCx territories, which also aligns with prior studies [7,8]. While this suggestion of missed disease is potentially worrisome, we were somewhat reassured by two observations. First, this non-significant increase in false negatives appeared to be much smaller for the test as a whole than it was for these two individual vessels. Second, there was no clinical suggestion of missed disease in the SPECT/CT cohort, given a lack of increase in the rates of death and MI, and a non-significant trend toward increase in the rate of clinically-indicated revascularizations. In parallel to the above changes in diagnostic accuracy, SPECT/CT reduced patient radiation exposure from MPI by 17 percent. This is in line with prior findings on the use of iterative reconstruction algorithms including RR and SC, which suggest that this technology enables reduction in radiotracer dose [11-14] without compromising diagnostic accuracy of the test [14]. While we cannot be certain which component of our system (CTAC, RR, or SC) was responsible for each of the above changes in diagnostic performance of SPECT MPI studies, we believe the overall effect on diagnostic accuracy with the new SPECT/CT system was favorable.
While the overall fraction of SPECT MPI patients referred for ICA within one year was not significantly altered, there was a decrease in the percentage of who underwent a purely diagnostic ICA procedure (8% vs. 3%; p = 0.01) and a non-significant increase in the percentage who underwent an ICA procedure leading to a clinically-indicated revascularization by PCI or CABG (7% vs. 4%; p = 0.26). This improvement in the clinical appropriateness of our ICA referrals appeared to most likely be a result of the more accurate risk stratification enabled by the updated SPECT/CT system. We feel this was a clinically meaningful improvement in quality.
In our study mean expenditures on preventable CAD-related healthcare services decreased during the year after MPI. These savings were primarily driven by the decrease in preventable diagnostic ICA procedures. Our study estimates that average savings on preventable healthcare expenses within the year following SPECT MPI is $1,452 per patient who received additional healthcare services. Averaged over all patients undergoing MPI (including those with no additional services), this equates to a savings of $443 per patient, which is more than 80% of the Medicare reimbursement for the original MPI study. If we assume that the cost of a new general purpose SPECT/CT system is around $1,000,000, then approximately 2,257 patients would need to be scanned in order for savings to equalize the upfront expense of a new SPECT/CT camera. At our medium-sized nuclear medicine practice, this represents approximately two years of MPI volume. The break-even point is even less if one considers only the cost difference between a new SPECT and a new SPECT/CT system. Currently the imaging provider absorbs the added expense of purchasing a new SPECT/CT system, while third-party payers reap these savings from decreased downstream costs. Our findings suggest that payers should consider directing patients preferentially to centers with SPECT/CT MPI technologies, or perhaps offer financial incentives for updating MPI imaging systems. Not only would this strategy save money at a systems level, but it would appear to prevent unnecessary invasive procedures.
Our study has several caveats. Our analysis of diagnostic accuracy is limited by looking only at clinically-indicated ICA procedures, which necessarily introduces a large referral bias toward patients with abnormal MPI studies. The overall prevalence of TP studies and FP will therefore be vastly inflated, while the incidence of FN studies will be reduced to an unknowable degree. The analysis of diagnostic accuracy is therefore useful only for comparison between groups, not for assessment of the test's diagnostic accuracy in any absolute sense. Also, since clinicians were not blinded to the fact that we had switched to use of CTAC, we cannot ignore the possibility of verification bias, rather than changes in diagnostic accuracy, affecting who was referred for ICA. The severity of stenosis on ICA was performed by visual assessment of angiographic severity which is inherently subjective by nature and measurement of fractional flow reserve (FFR) was not routinely performed. Our economic analysis is potentially limited by the decision to estimate from national average reimbursement data, rather than exact hospital record-based billing data; however, while this makes our cost estimates more theoretical, it should also make them more generalizable. Finally, it represents a single center's experience and the results need to be interpreted in that context. For example, our data may not be generalizable to centers with a larger volume of MPI studies, where purchasing a cardiac-only SPECT camera may be the most logical investment. We hope our financial analysis provides the impetus for others to evaluate how differences in MPI imaging technology (e.g. SPECT vs. SPECT/CT vs. PET) cause variability in downstream medical expenditures in large, multi-center cohorts. Hopefully, databases such as the American Society of Nuclear Cardiology Image Guide registry will allow for such analysis [23].
Conclusion
We found that upgrading from an older general-purpose SPECT camera with line-source attenuation correction to a new general-purpose SPECT/CT system with advanced image reconstruction algorithms resulted in overall improvements in diagnostic accuracy and a reduction in downstream preventable healthcare expenditures. While previous studies have shown similar effects on diagnostic accuracy, our data is the first to show that SPECT/CT has demonstrable economic benefits. Our findings suggest that the replacement of an older general-purpose SPECT camera with a general-purpose SPECT/CT system leads to meaningful quality improvement and is therefore a justifiable investment.
References
-
DePuey EG (2012) Advances in SPECT camera software and hardware: currently available and new on the horizon. J Nucl Cardiol 19: 551-581.
-
Heller GV, Bateman TM, Johnson LL, Cullom SJ, Case JA, et al. (2004) Clinical value of attenuation correction in stress-only Tc-99m sestamibi SPECT imaging. J Nucl Cardiol 11: 273-281.
-
Caobelli F, Akin M, Thackeray JT, Brunkhorst T, Widder J, et al. (2016) Diagnostic accuracy of cadmium-zinc-telluride-based myocardial perfusion SPECT: impact of attenuation correction using a co-registered external computed tomography. Eur Heart J Cardiovasc Imaging 17: 1036-1043.
-
van Dijk JD, Mouden M, Ottervanger JP, van Dalen JA, Knollema S, et al. (2016) Value of attenuation correction in stress-only myocardial perfusion imaging using CZT-SPECT. J Nucl Cardiol 1-7.
-
Masood Y, Liu YH, Depuey G, Taillefer R, Araujo LI, et al. (2005) Clinical validation of SPECT attenuation correction using x-ray computed tomography-derived attenuation maps: multicenter clinical trial with angiographic correlation. J Nucl Cardiol 12: 676-686.
-
Utsunomiya D, Tomiguchi S, Shiraishi S, Yamada K, Honda T, et al. (2005) Initial experience with X-ray ct based attenuation correction in myocardial perfusion SPECT imaging using a combined SPECT/ct system. Ann Nucl Med 19: 485-489.
-
Sharma P, Patel CD, Karunanithi S, Maharjan S, Malhotra A (2012) Comparative accuracy of CT attenuation-corrected and non-attenuation-corrected SPECT myocardial perfusion imaging. Clin Nucl Med 37: 332-338.
-
Genovesi D, Giorgetti A, Gimelli A, Kusch A, D'Aragona Tagliavia I, et al. (2011) Impact of attenuation correction and gated acquisition in SPECT myocardial perfusion imaging: results of the multicentre SPAG (SPECT Attenuation Correction vs Gated) study. Eur J Nucl Med Mol Imaging 38: 1890-1898.
-
Savvopoulos CA, Spyridonidis T, Papandrianos N, Vassilakos PJ, Alexopoulos D, et al. (2014) CT-based attenuation correction in Tl-201 myocardial perfusion scintigraphy is less effective than non-corrected SPECT for risk stratification. J Nucl Cardiol 21: 519-531.
-
Ou X, Jiang L, Huang R, Li F, Zhao Z, et al. (2013) Computed tomography attenuation correction improves the risk stratification accuracy of myocardial perfusion imaging. Nucl Med Commun 34: 495-500.
-
DePuey EG, Bommireddipalli S, Clark J, Leykekhman A, Thompson LB, et al. (2011) A comparison of the image quality of full-time myocardial perfusion SPECT vs wide beam reconstruction half-time and half-dose SPECT. J Nucl Cardiol 18: 273-280.
-
Ariel Gutstein, Roman Navzorov, Alejandro Solodky, Israel Mats, Ran Kornowski (2013) Angiographic correlation of myocardial perfusion imaging with half the radiation dose using ordered-subset expectation maximization with resolution recovery software. J Nucl Cardiol 20: 539-544.
-
Druz RS, Phillips LM, Chugkowski M, Boutis L, Rutkin B, et al. (2011) Wide-beam reconstruction half-time SPECT improves diagnostic certainty and preserves normalcy and accuracy: A quantitative perfusion analysis. J Nucl Cardiol 18: 52-61.
-
Lima R, De Lorenzo A, Camargo G, Oliveira G, Reis T, et al. (2014) Prognostic value of myocardium perfusion imaging with a new reconstruction algorithm. J Nucl Cardiol 21: 149-157.
-
Duvall WL, Croft LB, Ginsberg ES, Einstein AJ, Guma KA, et al. (2011) Reduced isotope dose and imaging time with a high-efficiency Czt SPECT camera. J Nucl Cardiol 18: 847-857.
-
Mouden M, Timmer JR, Ottervanger JP, Reiffers S, Oostdijk AH, et al. (2012) Impact of a new ultrafast CZT SPECT camera for myocardial perfusion imaging: fewer equivocal results and lower radiation dose. Eur J Nucl Med Mol Imaging 39: 1048-1055.
-
Songy B, Lussato D, Guernou M, Queneau M, Geronazzo R (2011) Comparison of myocardial perfusion imaging using thallium-201 between a new cadmium-zinc-telluride cardiac camera and a conventional SPECT camera. ClinNucl Med 36: 776-780.
-
Verger A, Imbert L, Yagdigul Y, Fay R, Djaballah W, et al. (2014) Factors affecting the myocardial activity acquired during exercise SPECT with a high-sensitivity cardiac CZT camera as compared with conventional Anger camera. Eur J Nucl Med Mol Imaging 41: 522-528.
-
Cerqueira MD, Weissman NJ, Dilsizian V, Jacobs AK, Kaul S, et al. (2002) Standardized myocardial segmentation and nomenclature for tomographic imaging of the heart. A statement for healthcare professionals from the Cardiac Imaging Committee of the Council on Clinical Cardiology of the American Heart Association. Circulation 105: 539-542.
-
Centers for Medicare and Medicaid Services Physician Fee Schedule Search.
-
Centers for Medicare and Medicaid Services Inpatient Charge Data Fiscal Year 2012.
-
Vollset SE (1993) Confidence intervals for a binomial proportion. Stat Med 12: 809-824.
-
Williams KA, McKinley AP, Executive Council of ASNC (2013) How the ASNC ImageGuide Registry will guide healthcare policy. J Nucl Cardiol 20: 948-950.
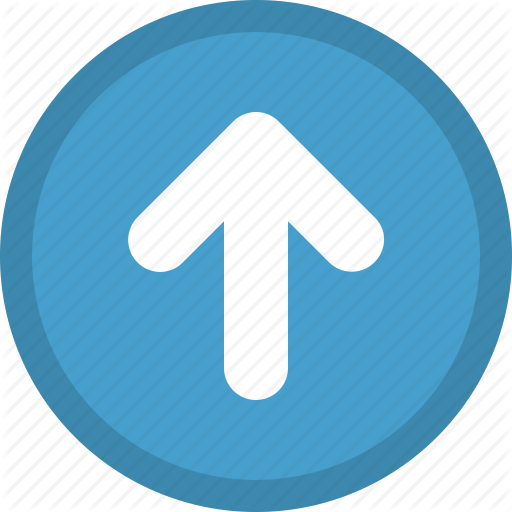